Natural fibers are an important source for producing polymers, which are highly applicable in their nanoform and could be used in very broad fields such as filtration for water/wastewater treatment, biomedicine, food packaging, harvesting, and storage of energy due to their high specific surface area. These natural nanofibers could be mainly produced through plants, animals, and minerals, as well as produced from agricultural wastes. For strengthening these natural fibers, they may reinforce with some substances such as nanomaterials. Natural or biofiber-reinforced bio-composites and nano–bio-composites are considered better than conventional composites. The sustainable application of nanofibers in agricultural sectors is a promising approach and may involve plant protection and its growth through encapsulating many bio-active molecules or agrochemicals (i.e., pesticides, phytohormones, and fertilizers) for smart delivery at the targeted sites. The food industry and processing also are very important applicable fields of nanofibers, particularly food packaging, which may include using nanofibers for active–intelligent food packaging, and food freshness indicators. The removal of pollutants from soil, water, and air is an urgent field for nanofibers due to their high efficiency. Many new approaches or applicable agro-fields for nanofibers are expected in the future, such as using nanofibers as the indicators for CO and NH3. The role of nanofibers in the global fighting against COVID-19 may represent a crucial solution, particularly in producing face masks.
1. Introduction
Nanotechnology has become one of the most promising research fields at the beginning of the 21st century due to its leading technology of the new industrial revolutions. Due to the growing environmental concerns of natural fibers, they have occupied a great position in the research community, which have many advantages such as recyclability, cheaper, high specific properties, lower density or lightweight, and biodegradability
[1,2][1][2]. Natural fibers also are considered renewable raw materials, which has become an obligatory issue for safe living
[3]. The synthetic material-based composites should be replaced by natural ones in several manufacturing industries, such as the field of textiles, which flax was used in ancient Egypt nearly 7000 years ago
[3]. The cellulose is the most important component of the lignocellulosic natural fibers, which many plants could order their content of cellulose (%) as follows straw of rice (41–57), leaf of date palm (46), leaf of abaca (56–63), bast of jute (61–71), leaf of banana (63–83), leaf of sisal (65), bast of hemp (68), bast of ramie (68.6–76.2), bast of flax (71), bast of kenaf (72), leaf of curaua (73.6), leaf of pineapple (81), bast of nettle (81–83), and seeds of cotton (83–91)
[2,3][2][3].
Nanofibers, as nanoproducts, have been used in several sectors, including biomedical, pharmaceutical, agricultural, and industrial fields. Nanofibers could be found in natural and synthetic form, whereas nanocellulose and its derivative materials are common natural fibers. Nanofibers have many advantages, such as large specific surface area, high porosity, and high size uniformity, which allow applying nanofibers in many environmental issues such as wastewater treatment and removing pollutants from soils
[1]. Nanofibers could be defined as nanostructures that may be fabricated using several methods such as the drawing method, template method, thermal-induced phase separation method, self-assembly, and electrospinning
[4]. The electrospinning method is considered the best method (simple and easy) to fabricate non-woven nanofibers, which could be used due to the high-molecular-weight polymers
[4]. Several applications of nanofibers have been confirmed, such as 3D printing of fiber-reinforced nanocomposites
[5], suitable nanocomposite materials and fiber-reinforced polymer for airplane manufacture
[6[6][7],
7], fiber nano–bio-compositions for cranioplasty, and other orthopedic applications
[8,9][8][9], replacing conventional rubber by the fiber-reinforced nanocomposites
[10], using nano–coconut shell filler mixed jute mat-reinforced epoxy composites for reducing the weight of the structures
[11].
2. Applications of Nanofibers in Agriculture
Recently, many researchers studied the main applications of nanofibers in agriculture because of their tailoring properties, including the biocompatible and biodegradable features, high surface area and porosity, ease of active ingredient additions (i.e., fungicides, insecticides, herbicides, pesticides, hormones, and pheromones), and flexibility of electrospun nanofibers
[55][12]. Nanofibers can apply for plant protection (through applying pesticides for pest control), plant growth (through applying hormones and/or fertilizers), pollution and contamination controls, and irrigation systems (through water filtration), as reported in
Table 31 by Meraz-Dávila et al.
[56][13], Raja et al.
[57][14], and
[58][15].
Table 31. The main applications of nanofibers in the agricultural sectors as reported by the literature.
Main Applications of Nanofibers in Agricultural Sectors |
References |
1—Nanofibers for good germination by coating seeds |
[59,60,61,62] | [16][17][18][19] |
2—Agro-wastes for production nanofibers |
[63,64] | [20][21] |
3—Nanofibers-based filters for irrigation systems |
[65] | [22] |
4—Nanofibers for plant protection |
[56] | [13] |
4.1 Encapsulation of fungicides |
[66,67] | [23][24] |
4.2 Encapsulation of herbicides |
[68] | [25] |
4.3 Detecting trace pesticides in water |
[69] | [26] |
5—Nano-silica grafted fiber |
[70] | [27] |
6—Smart nanotextiles for sustainable agriculture |
[13] | [28] |
7—Nanofibers for encapsulation of agrochemicals |
[71,72] | [29][30] |
7.1 Fertilizer application |
[73] | [31] |
7.2 Plant hormones (e.g., indole acetic acid) |
[57, | [14 | 74] | ][32] |
The main applications of nanofibers in the agricultural field may include coating seeds
[60,61,62][17][18][19], nanofibers-based filters for irrigation systems
[65][22], nanofibers for plant protection
[56][13] through encapsulation of fungicides
[66,67][23][24], or detecting trace some pesticides in water
[69][26], nano-silica grafted fiber
[70][27], smart nanotextiles for sustainable agriculture
[13][28], nanofibers for encapsulation of agrochemicals including fertilizer
[75][33], and phytohormones
[71,72][29][30]. Nanofibers can be used as a smart and sustained delivery of agricultural inputs through seed to improve germination and seedling growth in rice
[59,76][16][34] and cowpea
[60][17], groundnut
[57][14], and sesame
[62][19].
Nanofibers were applied for plant protection through encapsulation of pesticides
[56][13], including fungicides
[66[23][24],
67], herbicides
[68][25], nano-silica grafted fiber
[70][27], and smart nanotextiles for sustainable agriculture
[13][28]. The use of nanofibrous filters in irrigation systems may involve functionalization (i.e., adsorption, filtration, and sterilization) by bioactive compounds, which could be achieved by interfacial polymerization, doping nanoparticles, self-assembly, and surface coating cross-linking or grafting, layer-by-layer
[27][35]. Several nanomaterials such as graphene oxide could be used for water purification because of its multi-functionality, such as an antibacterial agent, excellent adsorption property, and photocatalytic abilities
[77][36]. Thus, nanofibers could be sustainably applied in many agricultural processes that lead to reduce the loss in used agrochemicals pesticides, hormones, and/or fertilizers
[57,61,62,63[14][18][19][20][21][22][23][24][25][26][27][29][30][31][32],
64,65,66,67,68,69,70,71,72,73,74], and to increase the productivity of crops through innovative management of phytopathogens or nutrients
[13][28].
3. Nanofibers for Water/Wastewater Treatment
Nanofibers are considered promising tools that are applied for diverse environmental conditions, especially polysaccharide-based electrospun nanofibers. The groups of polysaccharides are suitable materials for these environmental issues because of their biobased origins, variety of types, eco-friendly, and renewable nature
[78][37]. In general, the nanofibers have been applied for many environmental problems such as removing pollutants from the air by filtration
[79][38], water treatment
[80][39], antimicrobial treatment
[81][40], environmental sensing
[82][41], for heavy metal removing as adsorbents
[83[42][43],
84], and agricultural/environmental remediation
[78,85][37][44]. The environmental sustainability of water using cellulose nanofibers-based green nanocomposites is considered one of the most important environmental issues
[86][45].
Based on the potential of water treatments under the global water crisis in a pure and safe case, using nanofibers in this review in water/wastewater treatment and removing the pollutants is discussed in more detail as an urgent environmental task (
Table 42). The pollution of water causes an imbalance in different ecological environments and directly also affects human health. Thus, there is a great need for researchers to develop effective technology in wastewater purification
[35][46]. The main strategies in wastewater treatment may include filtration, adsorption, catalysis, centrifugation, biological treatment, and electro-coalescence
[87][47]. More than 200 natural and synthetic polymers were successfully electrospun into nanofiber membranes, such as polyimide (PI), polyacrylonitrile (PAN), poly/vinyl alcohol (PVA), poly/vinylidene-fluoride (PVDF), polylactic acid (PLA), cellulose acetate (CA), polyurethane (PU), polyethylene oxide (PEO), and polycaprolactone (PCL)
[35][46].
Table 42. Using of nanofibers in water/wastewater treatments for removing heavy metal pollutants.
Nanofibers and Their Average Diameter |
Max. Adsorption Capacity |
Pollutant |
References |
Polyvinylidene fluoride–polyacrylonitrile-ZnO nanofiber membranes (200 nm) |
350 mg g | −1 |
Cd |
[88] | [48] |
Amidoxylated polyacrylonitrile/Poly-vinylidene fluoride (AOPAN/PVDF) (235–314 nm) |
89.29 mg g | −1 |
Pb (II) |
[89] | [49] |
Nitro-oxidized carboxy-cellulose nanofibers obtained from moringa plants (0.22 µm) |
257.07 mg g | −1 |
Hg |
[90] | [50] |
Electrospun chitosan–polyethylene oxide-oxidized cellulose biobased composite (159.3 nm and 21.7 µm, resp.) |
15.72 mg g | −1 |
Cu |
[91] | [51] |
Modified poly butylene succinate nanofibers (10 µm) |
91.2 and 122 mg g | −1 | , respectively |
Ag (I) and Hg (II) |
[92] | [52] |
TEMPO-oxidized cellulose nanofibers (diameter 6.15 nm) |
56.50 mg g | −1 |
Cu (II) |
[93] | [53] |
Polyvinyl alcohol (PVP)-octa-amino-POSS nanofibers (21 µm) |
37.4 and 120 mg g | −1 | , respectively |
Cu (II), Pb (II) |
[94] | [54] |
Starch-g-poly(acrylic acid)-cellulose nanofiber bio-nanocomposite hydrogel (10 µm) |
40.65 mg g | −1 |
Cd (II) |
[95] | [55] |
Oxidized regenerated cellulose nanofiber membrane (10 µm) |
20.78 and 206.1 mg g | −1 | , respectively |
Cu (II), Pb (II) |
[96] | [56] |
polyvinylidene fluoride–amidoximized polyacrylonitrile nanofibers (20.7 µm) |
30.1, 25.8, and 72.5 mg g | −1 | , respectively |
Cu (II), Ni (II), Pb (II) |
[97] | [57] |
Modified prepared polyacrylonitrile nanofibers (320 nm) |
22.95 and 12.36 mmol g | −1 | , respectively |
Cu and Pb |
[98] | [58] |
Centrifugal spinning of lignin amine/cellulose acetate nanofiber (756 nm) |
50.08 and 31.17 mg g | −1 | , respectively |
Cu (II), Co (II) |
[83] | [42] |
Visualized chitosan–polyacrylonitrile nanofiber membrane |
164.3 mg g | −1 |
Cu (II) |
[99] | [59] |
Zn/Al/gallate layered double hydroxide–polystyrene nanofibers (2–5 µm) |
190 mg g | −1 |
Cu (II) |
[100] | [60] |
Polyacrylonitrile–polyetherimide nanofibers (0.84 mm) |
242.7, 214.1, 258.3 mg g | −1 | , respectively |
Cu (II), Cr (VI), As (V) |
[101] | [61] |
Concerning the mechanism of cellulose nanofibers (CNFs) in water purification, CNFs can make a link with carboxylic surface functional groups by oxidation and chemically bonded nanocomposites based on modified CNFs with various metal–organic or metal pillars frameworks in order to create a robust and high-efficiency material
[86][45]. The mechanism of water purification using nanofibers consists of both physical and chemical methods that could be explained based on the chemical and physical bases (
Figure 1). Chemically, the formation of stable chemical bonds between nanofibers and metal ions and relevant oxidation-reduction is involved. Physically, the surface area and pore volume of nanofibers are key parameters determining the fiber adsorption capacity and, therefore, water treatment performance. This mechanism was confirmed by many researchers, such as Agrawal et al.
[102][62] and Uddin et al.
[103][63]. Many recent reviews were published on the removing of hazardous pollutants (i.e., both organic and inorganic materials) from water/wastewater using nanofibers such as Chen et al.
[104][64], Cui et al.
[35][46], Ibrahim et al.
[105][65], Jahan and Zhang
[106][66], Marinho et al.
[107][67], Sakib et al.
[80][39], Sjahro et al.
[108][68], and El-Aswar et al.
[109][69]. These previous studies confirmed that electrospun nanofiber membranes could be easily used for achieving different water treatments by combining multifunctional materials due to their high specific surface area and unique interconnected structure
[104][64].
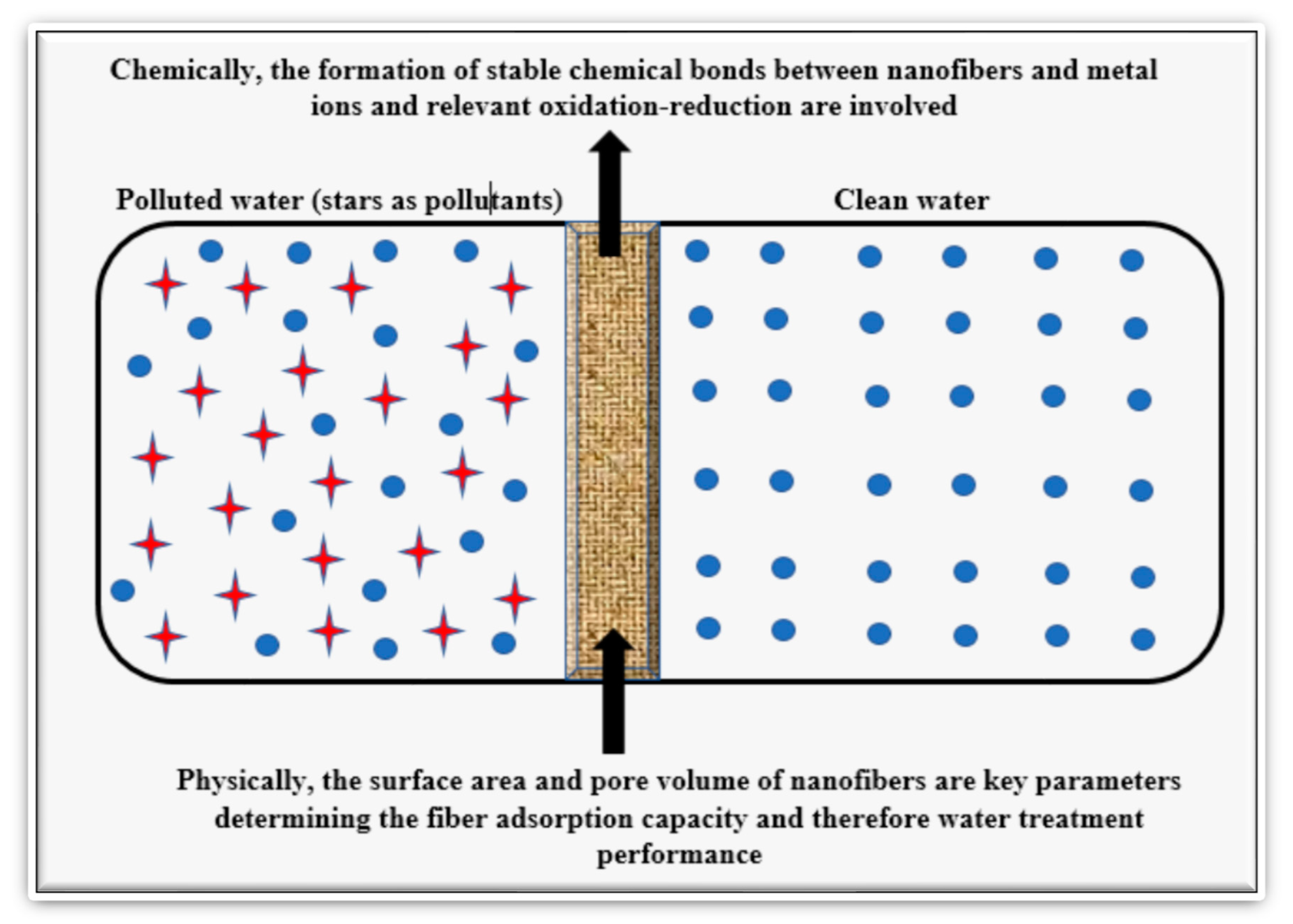
Figure 1. The mechanism of water purification using nanofibers consists of both physical and chemical methods, which illustrates electrostatic and intermolecular forces between nanofibers and pollutants in water.
4. Conclusions
A comparison between natural fibers and nanofibers was discussed in this review, besides different sustainable applications of nanofibers in fields of biomedicine, agriculture, and the environment. Producing nanofibers from different agro-wastes and applying nanofibers for food packaging were also the main topics in this manuscript. Based on the environmental problems of synthetic nanofibers, particularly petroleum-based fiber composites, natural nanofibers are recommended especially after reinforcement, along with the matrix, for more performance and strength of the composites. The nano–bio-composites could produce by coupling matrix of nanoparticles into bio-reinforcer, which converted into the biofiber-reinforced polymer matrix. Nano-based reinforced polymeric composites can be applied for sophisticated applications, mainly in agriculture (irrigation system, seed coating, for plant protection, agrochemical encapsulation), environmental (removing pollutants from the air by filtration, antimicrobial treatment, environmental sensing, for heavy metal removing as adsorbents, and agricultural/environmental remediation, and wastewater treatment), and food sectors (food packaging, beverage industry, encapsulation of food materials, food preservation, and nanofibers for electrochemical DNA biosensors). From the previous applications, using nanofibers in water treatment and food packaging are important areas for research and development.