Peroxisome Proliferator-Activated Receptors (PPARs), belonging to the nuclear receptor superfamily, are transcription factors playing well-established roles in several metabolic pathways in the organism, including lipid and glucose metabolism, energetic homeostasis, cell differentiation and proliferation. Since their discovery, a body of knowledge has been collected on them, and the three receptor subtypes (PPARα, PPARγ, PPARβ/δ) have attracted a great deal of efforts by medicinal chemists to identify novel drugs targeting the metabolism. PPAR agonists, antagonists, and modulators represent important pharmacological tools to induce beneficial therapeutic effects in metabolic disorders, such as metabolic syndrome, obesity, diabetes, and others.
1. Introduction: Targeting Metabolism during Viral Infections
The viral reproduction needs the host cell machinery for the synthesis of viral components, such as nucleic acids and proteins. Clathrin-mediated endocytosis or micropinocytosis are the mechanisms allowing the internalization of viruses in the host, which are subsequently released in the cytosol. At this stage, the viral genome is released and transported into sites where the viral replication occurs
[1]. After the replication of viral genome and the subsequent assembly of novel particles (virions), virus leaves from the host cell by exocytosis or lysis
[2]. The metabolic capacity of the host cell is of primary importance to ensure the formation of novel virus particles, by supplying the necessary metabolites as amino acids, fatty acids, and nucleotides. Many viruses can manipulate the host metabolism to optimize their biosynthetic needs. The multiple effects played by viruses, involving glucose, lipid, and glutamine metabolism, were extensively reviewed by Eisenreich et al.
[3]. The understanding of the nature of metabolic changes imposed by a viral infection is of paramount importance to identify a targeted therapy and obtain a successful outcome. For example, during the human immunodeficiency virus (HIV) infection, the inhibition of glucose metabolism determines the viral elimination, while during the late stages of HIV infection, the intervention on lipid metabolism has been proposed as a fruitful antiretroviral strategy
[4,5][4][5]. Interestingly, the nutrition status plays a key role in influencing the outcome of viral infections: it is well known that malnutrition, especially a marked reduction in calorie intake, increases the susceptibility of children to viral diseases. Several studies have been conducted in animals to analyze the effects of diet supplementation, mainly vitamins, fat, fibers, and amino acids, during viral infections
[6,7,8,9][6][7][8][9]. Several metabolic strategies have been proposed to target the viral replication in cell cultures and in vivo models.
Figure 1 summarizes the main metabolic approaches pursued to manipulate virus metabolism, affecting the glucose, lipid, and glutamine metabolic pathways.
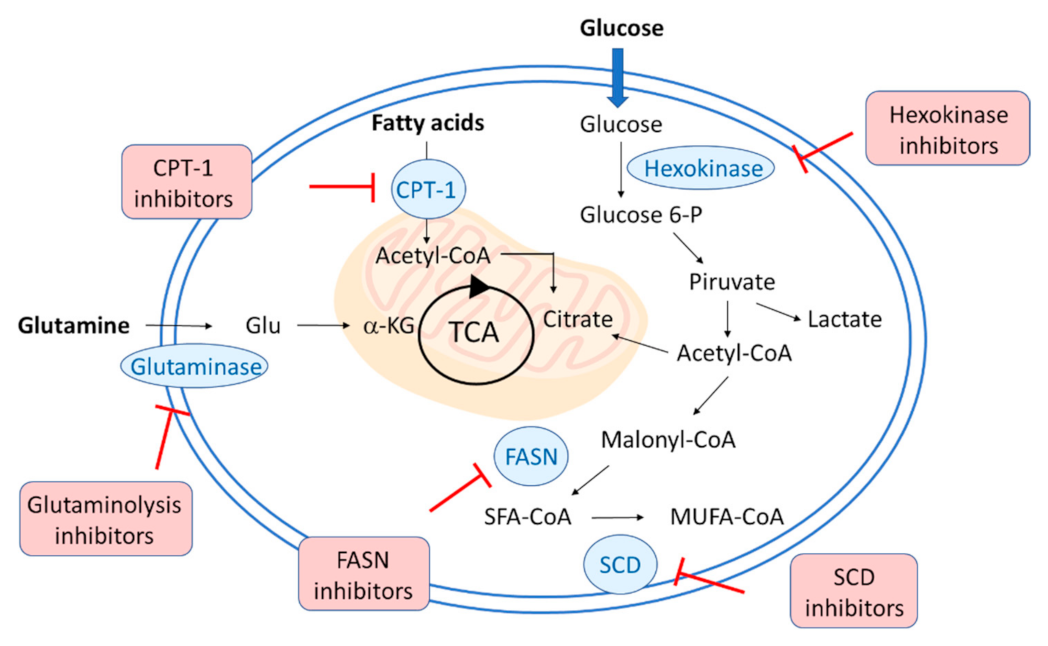
Figure 1. Schematic representation of the metabolic strategies to target viral replication. Hexokinase inhibitors interfere with glycolysis and glucose metabolism, whereas glutaminolysis inhibitors block the glutamate formation from glutamine. The lipid metabolism can be affected with different strategies: carnitine palmitoyl transferase 1 (CPT1) inhibitors block the entry of long-chain fatty acids into mitochondria for oxidation, fatty acid synthase (FASN or FAS) and stearoyl-CoA desaturase (SCD) inhibitors strongly interfere with lipogenesis.
Among several metabolic compounds tested for their antiviral activity, promising results were obtained with 2-deoxy-D-glucose (2-DG), which targets glucose metabolism, C75 and MK8245, which interferes with fatty acid metabolism, and CB-839, with its action on glutamine metabolism (
Figure 2).
Figure 2. Chemical structures of selected metabolic drugs tested to contrast the virus replication: 2-deoxy-D-glucose (2-DG, phosphoglucose isomerase inhibitor), C75 (FAS inhibitor), MK8245 (SCD1 inhibitor), and CB-839 (glutaminase inhibitor).
2-DG is a synthetic glucose analog in which the 2-hydroxyl group is replaced by hydrogen. It undergoes an in vivo phosphorylation to 2-deoxy-D-glucose-6-phosphate, which remains trapped into cells, being unable to form its isomer fructose-6-phosphate. The intracellular accumulation of 2-deoxy-D-glucose-6-phosphate leads to the inhibition of glycolysis and glucose metabolism. 2-DG has been attracting interest also as a potential anticancer molecule, thanks to its non-toxic profile and its oral bioavailability
[10,11,12][10][11][12]. The influence of 2-DG was studied in different viral infections, such as herpes simplex virus (HSV)
[13], influenza virus
[14], cytomegalovirus
[15], rhinovirus
[16]; very recently it has been proposed as an adjuvant therapeutic agent for COVID-19 treatment due to its effects on glycolysis, anti-inflammatory action, and interaction with viral proteins
[17].
2. PPARs in Viral Infections
Recently, PPARs have been attracting a great deal of interest as targets in different cancers, thanks to their ability to induce important metabolic changes in tumors, and several PPAR ligands have been studied for their possible antitumor effects
[57,58,59,60][18][19][20][21].
The involvement of PPARs in viral infections is quite a recent matter of investigation, and the interest in this field received a strong impulse due to the pandemic by COVID-19. PPAR agonists, antagonists, or modulators could induce different effects in models of infections; in the following sections, the main results obtained from in vitro and in vivo studies will be analyzed and discussed. In addition, PPAR modulators may act as agonist or antagonist depending on the cellular context, based on the variable presence of coactivators or corepressors in specific tissues. Unfortunately, it is currently impossible to precisely categorize the effects of PPAR ligands in viral infections, so as identify the exact mechanism of action responsible for the observed antiviral outcome. In next paragraphs main evidence on PPAR involvement in the most studied infections (SARS-CoV-2, HCV, and HCMV) will be analyzed, focusing on the description of PPAR ligands and the beneficial effects observed in models of infection.
2.1. Antiviral Effects Played by PPAR Ligands in SARS-CoV-2 Infection
The COVID-19 pandemic is an ongoing global problem caused by severe acute respiratory syndrome coronavirus 2 (SARS-CoV-2). A very large body of studies has been conducted on this subject, with a recent investigation demonstrating that cell lipid profile is significantly altered during the infection by human coronavirus
[61][22]. COVID-19 progression has been suggested to have a metabolic origin, while elevated glucose and lipid levels are risk factors. The SARS-CoV-2 spike protein mediates the viral cellular entry via the ACE2 receptor. Recent findings have revealed that ACE2 is downregulated in SARS-CoV-2-infected lung tissue
[62][23]. The stimulation of ACE2 could play a protective role in the treatment of hypertension, heart disease, cancer, and COVID-19
[63][24], while these disorders show the upregulation of the WNT/β-catenin pathway. For these reasons, pharmacological agents increasing the ACE2 expression, such as statins and PPARγ agonists, could be beneficial in the treatment of COVID-19.
In Figure 3 are summarized the chemical structures of natural and synthetic PPAR ligands explored in in vitro assays or proposed as possible antiviral agents against SARS-CoV-2 infection.
Figure 3. Chemical structures of natural and synthetic PPAR ligands explored in in vitro assays or proposed as possible antiviral agents against SARS-CoV-2 infection.
In
Table 1 results from published studies are summarized, pointing at PPAR ligands, concentration, infected cell models, and observed outcome in SARS-CoV-2 infection. In addition, PPAR ligands proposed as anti-SARS-CoV-2 agents are reported, based on their anti-inflammatory, antioxidant, and immunomodulating properties.
Table 1.
Summary of PPAR ligands in vitro tested in SARS-CoV-2 infection or proposed as anti-SARS-CoV-2 agents.
Cpd |
PPAR Activity |
Concentration |
Cell |
Outcome |
Ref. |
Fenofibrate (Tricor | ® | ) |
PPARα agonist |
20 µM |
Human bronchial epithelial cells |
Block viral replication, reverted effects on phospholipid accumulation and glycolysis |
[65] | [25] |
Fenofibrate |
PPARα agonist |
20 µM |
Vero E6 cells |
Block viral entry |
[66] | [26] |
Palmitoylethanolamide |
PPARα agonist |
10 | −9 | –10 | −7 | M |
murine alveolar macrophages |
Inhibition of NF-κB transcription and NLRP-3 inflammasome signaling, with a significant antinflammatory effect |
[67] | [27] |
Pioglitazone |
PPARγ agonist |
- |
- |
Proposed as 3CL-Pro inhibitor, it could downregulate SARS-CoV-2 RNA synthesis and replication Proposed as an additive in COVID-19 patients with diabetes, hypertension, and cardiovascular comorbidities for its antinflammatory properties |
[71,72] | [28][29] |
Curcumin, capsaicin, docosahexanoic acid, eicosapentaenoic acid |
Natural PPARγ agonists |
- |
- |
- |
Proposed for use in COVID-19, due to its anti- inflammatory and antioxidant properties |
[ | 73 | ] | [31] |
Astaxanthin |
Multiple action on PPARs: PPARα agonist, PPARδ antagonist, PPARγ agonist or antagonist |
- |
- |
Proposed for use in COVID-19, due to the ability to reduce the oxidative stress induced by ROS, the immune response, and the production of pro-inflammatory cytokines |
[74] | [32] |
2.2. Antiviral Effects Played by PPAR Ligands in HCV Infection
The role of PPARs in controlling glucose and lipid metabolism in hepatocytes is well established, leading to liver inflammation and fat accumulation, which represent hallmarks of chronic HCV infection. To date, many studies have been carried out to clarify the exact correlation between PPARs and HCV infection
[80][33]. PPARs are highly expressed in hepatocytes in normal conditions, whereas it has been demonstrated an altered liver PPARα and γ expression during HCV infection
[81,82,83][34][35][36]. The correlation between PPARα expression and HCV attracted a great deal of attention, this isoform being a major player in the liver lipid homeostasis.
In
Figure 4, chemical structures of natural and synthetic PPAR ligands tested in in vitro or in vivo studies against HCV infection are displayed.
Figure 4. Chemical structures of natural and synthetic PPAR ligands displaying in vivo or in vitro efficacy in HCV infection models.
A summary of PPAR ligands tested in in vivo or in vitro HCV infection models is depicted in
Table 2.
Table 2.
Summary of PPAR ligands in vivo or in vitro tested in HCV infection.
Cpd |
PPAR Activity |
Concentration |
Model |
Outcome |
Ref. |
Bezafibrate |
PPAR pan agonist |
400 mg/day for 8 weeks |
Chronic hepatitis C patients complicated with hyperlipidemia |
Decreased serum HCV RNA |
[86] | [37] |
Pioglitazone |
PPARγ agonist |
30 mg/day for 14 days |
Overweight Genotype 4 HCV patients |
Decreased serum HCV RNA at day 14 |
[87] | [38] |
Naringenin |
PPARα agonist |
200 µM |
HCV-infected Huh7.5.1 |
Inhibition of ApoB-100 and HCV RNA secretion |
[88] | [39] |
Fluoxetine |
PPARγ/δ modulator |
0.1–10 µM for 6 days |
HCV-infected Huh7.5 cells |
Decrease in virus protein levels of core, NS3, and NS5A. Reduction in ROS generation and lipid accumulation |
[89] | [40] |
- |
Calcitriol |
PPARα/γ/δ modulator |
0.1–1000 nM |
HCV-infected Huh7.5 cells | Proposed for use in COVID-19, due to their ability to prevent cytokine overproduction and inflammatory cascade |
[69] | [ |
Decrease in viral infection, nitrative stress, and lipid accumulation30] |
[ | 90 | ] | [ | 41 | ] |
Gamma-oryzanol |
T0070907 | PPARγ modulator |
PPARα/γ antagonist |
IC | 50 | 19.1 µM |
Huh-7 cells expressing an HCV subgenomic replicon |
Inhibition of HCV replication |
[91] | [42] |
Biphenylcarboxylic acids |
PPARδ antagonists |
2.5–10.0 µM, most potent compound EC | 50 | 0.22 µM |
OR6 HCV replication system |
Dose-dependent inhibition of HCV RNA replication. Synergistic antiviral effect when tested in combination with Peg-IFNα or Peg-IFNα and ribavirin |
[92] | [43] |
2.3. Antiviral Effects Played by PPAR Ligands in HCMV Infection
Although infection by HCMV is usually benign, congenital infection is a leading cause of permanent abnormalities of the CNS. In addition, HCMV infection contributes to the development of pathological states in immune-compromised hosts such as AIDS patients and transplant recipients. The relationships between HCMV infection and PPARs have been explored, given the known role of PPARs, mainly PPARγ isotype, in lipogenesis and inflammation. Experiments provided evidence that PPARγ transcriptional activity is induced in HCMV-infected cells, as evidenced by nuclear translocation and lipid accumulation
[93][44]. The PPARγ activation by HCMV is involved in defective trophoblastic migration and invasion processes, being PPARγ a major player in cytotrophoblast differentiation and function.
The effects of HCMV infection in human immortalized extravillous cytotrophoblasts (HIPEC) were also analyzed, identifying 15-hydroxyeicosatetraenoic acid (15-HETE) and 13-hydroxyoctadecadienoic acid (13-HODE) as predominant PPARγ agonists secreted after HCMV infection
[94][45]. The PPARγ stimulation by these natural ligands results in impaired migration abilities and enhanced viral replication, confirming the important role of PPARγ in HCMV infection.
In addition, the role of PPARγ in neurogenesis during congenital HCMV infection was deeply studied in neural stem cells from human embryonic stem cells (NSCs)
[95][46]. This study confirmed a key role for PPARγ in neurogenesis and in the pathophysiology of HCMV infection, displaying that viral infection triggers PPARγ levels in NSCs. Infected cells produce increased levels of 9-hydroxyoctadecadienoic acid (9-HODE), which is responsible for enhanced viral replication and impaired neurogenesis. The treatment of infected NSCs with the PPARγ antagonist T0070907 restored a normal rate of differentiation.
To date, only the relationship of HCMV-PPARγ has been reported in the literature, and details about the use of PPARγ agonists or antagonists are not available. To the best of our knowledge, no studies have been published focusing on the possible effects played by PPARα and PPARδ pathways during HCMV infection.
3. Conclusions
The strict interplay existing between virus and host metabolism offers the possibility to interfere with metabolic pathways as an attractive novel strategy to obtain a successful outcome in viral infections. The possibility to target PPARs has received attention in recent years, given the well-established roles of these nuclear receptors in glucose and lipid metabolism. However, to date, a limited number of experimental studies describing PPAR ligands tested in viral infections are available, and they mainly involve SARS-CoV-2, HCV, and HCMV infections. These studies were reviewed in this work, pointing at chemical structures, mechanisms of action on three PPAR isoforms (agonist, antagonist, modulator), and the outcomes observed in in vitro and in vivo models of infection. Unfortunately, it is currently impossible to precisely categorize the effects of PPAR ligands in viral infections, so as to identify the exact mechanism of action responsible for the observed antiviral effects. In SARS-CoV-2 infection, the use of PPAR ligands could induce beneficial effects by preventing the cytokine overproduction and the subsequent inflammatory cascade. Additionally, in HCV, the use of PPAR agonists and antagonists was found to be effective to decrease viral levels in patients or in in vitro cell models. Very little is known about the possibility to use a PPAR ligand to contrast the HCMV infection; however, some studies provide evidence about the relationships between PPARs and HCMV infection, suggesting PPAR ligands as therapeutic options in the management of HCMV-infected patients. A deeper understanding of viral interactions with host metabolism is necessary to provide novel therapeutic options in the fight against viral diseases. A further aspect to be clearly elucidated is to know the exact mechanism of action of PPAR drugs, excluding possible off targets effects.