The photooxidative degradation process of plastics caused by ultraviolet irradiation leads to bond breaking, crosslinking, the elimination of volatiles, formation of free radicals, and decreases in weight and molecular weight. Photodegradation deteriorates both the mechanical and physical properties of plastics and affects their predicted life use, in particular for applications in harsh environments. Plastics have many benefits, while on the other hand, they have numerous disadvantages, such as photodegradation and photooxidation in harsh environments and the release of toxic substances due to the leaching of some components, which have a negative effect on living organisms. Therefore, attention is paid to the design and use of safe, plastic, ultraviolet stabilizers that do not pose a danger to the environment if released. Plastic ultraviolet photostabilizers act as efficient light screeners (absorbers or pigments), excited-state deactivators (quenchers), hydroperoxide decomposers, and radical scavengers. Ultraviolet absorbers are cheap to produce, can be used in low concentrations, mix well with polymers to produce a homogenous matrix, and do not alter the color of polymers.
1. Introduction
Ultraviolet (UV) light has harmful effects on materials used in outdoor applications. Plastics suffer photooxidation when exposed to harsh conditions (high temperature, sunlight for long duration, and humidity) in the presence of oxygen. Plastic degradation, as a result of UV light absorption, leads to discoloration, cracks, and loss of mechanical and physical properties
[1][2]. Photooxidation resembles autooxidation due to long-term heat aging, except that the driving force is UV light and not heat
[3]. Therefore, during plastic manufacturing, measures should be taken to ensure that the materials will last longer and to inhibit photooxidation and photodegradation processes.
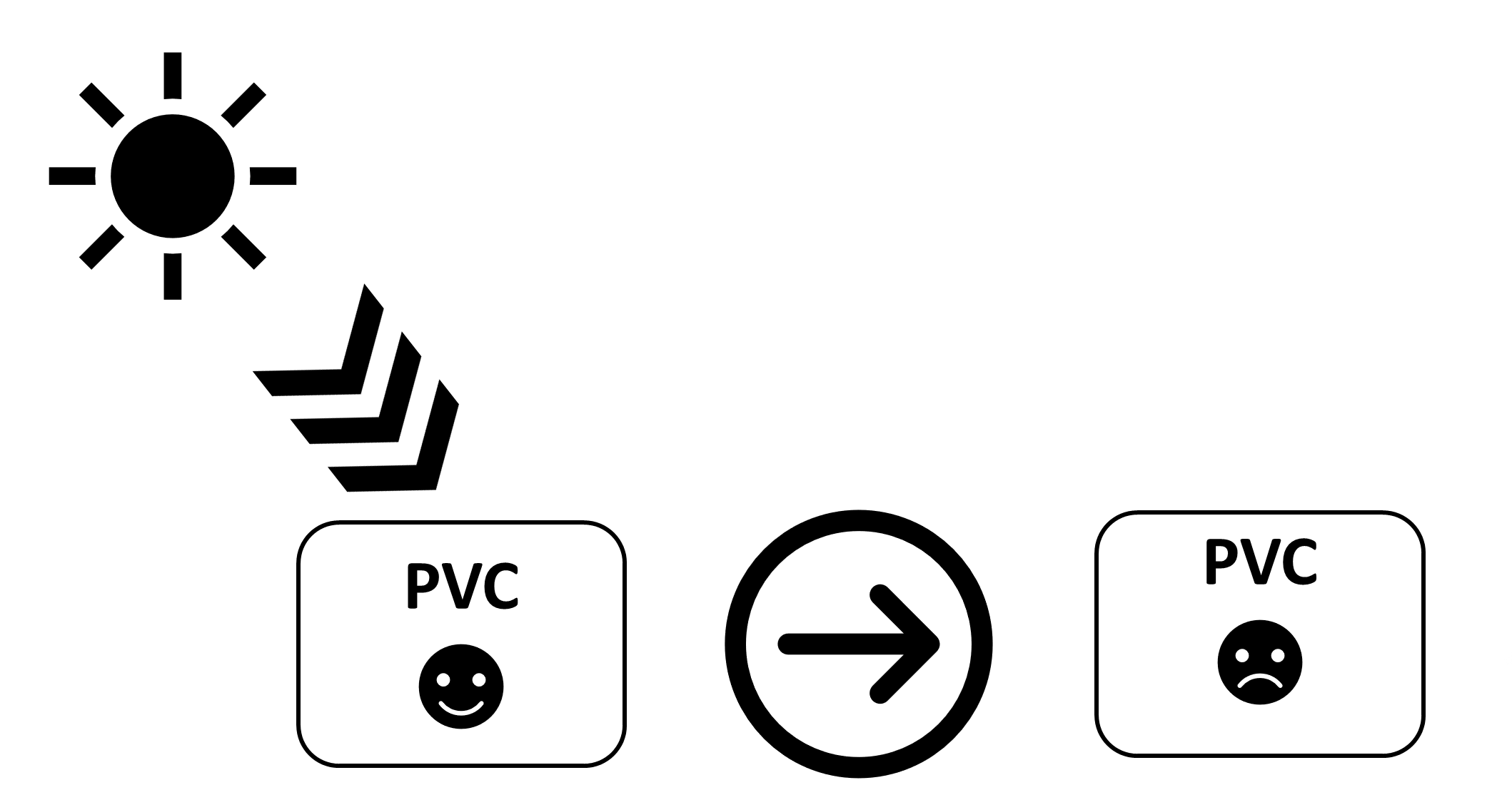
The polymerization technique was developed over the years to allow the production of plastics on an industrial scale. There has been a massive increase in the production of plastics in recent years
[4]. The scale of polyvinyl chloride (PVC) production has increased over the years from 3 million tons in 1965 to over 40 million tons in 2018 and is expected to grow further to 60 million tons in 2025
[5]. PVC can be produced in different forms and shapes, using both suspension and emulsion polymerization
[6]. Plastic waste is a challenge, and there is a need for not only effective recycling but cutting the waste at the source. Therefore, further developments in plastic are still needed to keep the environment clean and to elongate the lifetime of plastic
[7][8].
Plastic contains polymeric chains that are based on carbon, hydrogen, and heteroatoms (e.g., sulfur, oxygen, or nitrogen). Polystyrene (PS), polypropylene (PP), polyethylene (PE), PVC, polyethylene terephthalate (PET), and polyurethane (PU) represent 75–80% of Europe’s plastic consumption (
Table 1)
[9][10]. These polymers have either C–C or C–heteroatom backbones, and their properties are highly dependent on the repeating units
[11].
Plastics are highly involved in our daily lives, from household items to very complex medical equipment. They are used in construction materials (e.g., windows, panels, glazing, coatings, siding, roofing, flooring, fencing, and decoration), furniture, offices, agriculture (e.g., mulch film, materials for greenhouses, and production of sacks), transportation (e.g., bodywork and production of protective coatings), flame and smoke retardants (high content of chlorine; 57% by weight), insulators, and others
[6]. Polycarbonate plastic has a low thermal conductivity (k) and, therefore, is better than conventional glazing agents
[12]. The demand for plastic has extensively increased due to its unique mechanical and physical properties (e.g., light weight, strength, resistance to corrosion and chemicals) and low manufacturing cost. In addition, the shape and properties of plastics can be manipulated based on the application. However, UV radiation has a negative effect on plastic (e.g., rigid PVC) lifetime and leads to the loss of its strength. The solar irradiation of PVC causes discoloration and the emission of toxic volatiles, which hinders its use in outdoor applications
[13]. PVC is still used as a construction material, but it can be replaced by polyolefins, which are less harmful but cost more.
The degradation of plastics is of major concern from an environmental perspective in terms of potential hazards to living organisms. The degradation of plastics takes place under either abiotic or biotic (e.g., biodegradation) conditions
[14]. Biodegradation is highly dependent on environmental factors, which vary based on the type of polymer. Color changes and the crazing of plastic are early signs of degradation, followed by surface cracking and the formation of small fragments
[15]. Floating plastics in seas and oceans are moderately affected by temperatures, solar radiation, and oxygen through photoinitiated oxidative degradation. For abiotic degradation, the contributing factors are sunlight and oxygen, and they affect the plastic through a hydrolysis process
[16].
Three steps (initiation, propagation, and termination) are involved in plastic degradation
[17]. The first step is initiated through solar or thermal initiators and leads to the formation of free radicals. Photoinitiation is not likely for both PE and PP, since they do not have unsaturated chromophores in their skeletons that are responsible for the absorption of light
[18]. Impurities or abnormalities within plastics allow for the production of free radicals leading to C–H bonds cleavage in the backbone of polymers
[19][20]. In the presence of oxygen, free radicals produce peroxy reactive moieties in the propagation step. In addition, hydroperoxides can be produced, leading to the autoxidation of polymers
[21]. In the propagation step, crosslinking or chain scission takes place
[22]. The deactivation of free radicals occurs in the termination step, leading to stable products. In the presence of oxygen, the formation of oxygen-containing moieties is expected, which leads to a photoinitiated degradation process. The chain scission and crosslinking (termination) of oxygenated species leads to the formation of olefins (unsaturated polymeric chains), aldehydes, and ketones (
Figure 1)
[23].
Figure 1. Abiotic degradation pathways for PE (R = H), PP (R = Me), and PS (R = Ph).
Plastic natural degradation is initiated through photodegradation followed by thermo-oxidative degradation
[24]. Sun UV light provides the energy needed to initiate the incorporation of oxygen into the polymeric chains
[25]. Plastics are degraded to small polymeric fragments and then metabolized by microorganisms in the surrounding environment. Microorganisms tend to convent the polymeric chain carbons to either carbon dioxide or biomolecules
[26][27]. However, such a process is very slow (taking up to 50 years) for the complete degradation of plastics
[28]. Chromophores present within the skeleton of polymers absorb visible or UV light, and therefore initiate the photodegradation process
[29][30]. Photodegradation takes place either in the presence of oxygen (e.g., photooxidation) or in its absence (e.g., chain crosslinking or bond breaking). When polymers (e.g., polyolefins) are exposed to heat, UV light, or mechanical stress in the presence of oxygen, they produce free radicals that initiate the oxidation process. Therefore, plastics should be stabilized to inhibit the oxidative processes to increase the half-life time of materials
[31].
Plastic weathering involves changes in the physical, mechanical, and chemical properties of polymers, particularly at the surface
[32]. Solar energy, moisture (e.g., rain, snow, or humidity), oxidants (e.g., ozone or atomic or singlet oxygen), and air pollutants (e.g., sulfur dioxide, nitrogen oxides, or polycyclic hydrocarbons) are responsible for these changes
[33]. Uneven discoloration, surface cracks, or loss of strength are the most common changes within plastics due to degradation
[34]. Climate change and the rise in global temperatures accelerate polymers’ weathering, and impurities (e.g., traces of metals or oxidants) present in additives increase the rate of photodegradation
[35].
PVC is a synthetic plastic that is similar to PP, but the backbone carbons are attached to chlorine atoms instead of hydrogens. PVC is one of the most common manufactured plastics
[36]. Due to the high content of chlorine, PVC is hard and stiff. In addition, PVC is polar due to the presence of C–Cl bonds and is soluble in many solvents, particularly those containing polar atoms such as ethers (e.g., dioxane, tetrahydrofuran, ketones, or nitrobenzene). It has a low cost, is durable, has excellent performance, is easily molded, and can be obtained in different shapes that are suitable for many applications. PVC is commonly used in packaging, health care devices, toys, construction materials, electrical wire insulation, clothes, and furnishing
[5][6]. For outdoor applications, PVC photostability should be enhanced through the addition of suitable additives to inhibit its photodegradation. The dechlorination of PVC is autocatalytic, which leads to the formation of –C=C–. The formation of unsaturated double bonds within the backbone of PVC leads to its photodegradation, in which small fragments and polyene residues are produced (
Figure 2)
[37].
Figure 2. Dechlorination of PVC and formation of polyene polymeric chains.
Plastic recycling has received attention recently due to the large volume of waste that it generates
[38]. Pyrolysis and incineration of PVC are not recommended due to the high level of hydrogen chloride (HCl) and other toxic volatiles produced
[39]. The most common methods for PVC recycling include chemical and mechanical techniques. Mechanical recycling is preferred when the PVC waste composition is known
[40]. On the other hand, the chemical recycling of PVC converts plastics back to chemicals that can be reused in the polymerization process. The development of techniques and instrumentation for the separation of PVC from the waste stream is still important to allow for the recovery of most wasted PVC.
2. Photostabilization of Polymers Using UV Absorbers
UV absorbers play an important role in absorbing harmful radiation from light and dissipating it as harmless thermal energy
[41][42][43]. In addition, they block the formation of free radicals that are produced at the early stages of degradation. The most common industrial UV absorbers are titanium oxide, carbon black, benzophenones, and triazoles (e.g., hydroxylbenzophenone and hydroxyphenylbenzotriazole), while the most common additives used recently for research include Schiff bases and organometallic complexes (
Figure 3).
Figure 3. Structures of some common UV absorbers.
These additives have unique UV absorbance characteristics. For example, benzophenone-containing additives absorb UV strongly in the short-wavelength region through a proton transfer or tautomeric structure equilibrium (
Figure 4). They are more efficient compared with the additives containing benzotriazole. Benzophenone-based UV absorbers have unique properties such as low cost, low toxicity, and good resistance to water and acids
[44].
Figure 4. Hydroxybenzophenones energy dissipation through a proton transfer.
Triazoles have high molar extinction coefficients (5 × 10
6 cm
−1M
−1) and absorb the most destructive wavelength of light (280–370 nm), which is highly involved in polymer degradation. The excitation of benzotriazoles takes place once the UV light is absorbed; the benzotriazoles then dissipate the energy through either heat release, involving a hydrogen transfer, or fluorescence emission
[45]. In addition, UV absorbers act as quenchers (Q) for the triplet excited state of the polymer chromophoric group (P *), followed by the release of energy as harmless heat (
Figure 5)
[46]. Similarly, metal complexes act as effective UV quenchers due to their low excitation coefficients and quench the triplet state of the carbonyl groups in polyolefins
[47][48][49].
Figure 5. UV absorbers act as quenchers for the excited state energy of polymers. * Represents the excited state.
Recently, we synthesized a range of UV stabilizers (e.g., aromatics, heterocycles, Schiff bases, organometallic complexes, and polyphosphates) and tested their efficiency for the stabilization of polymers
[50][51][52][53][45][54][55][56][57][58][59][60][61][62][63][64][65][66][67][68][69][70][71][72][73][74][75][76][77][78][79][80][81][82][83][84]. These additives, at a low concentration of 0.5% by weight, led to a significant improvement in the photostability of polymers. The stabilization effect that the UV absorbers induced in polymers was examined using infrared spectroscopy, the determination of weight and molecular weight, and inspection of the surface of polymers.
In conclusion, polymer stabilization is one of the most important processes that is used to elongate the lifetimes of plastic products. Plastics used in outdoor applications suffer in harsh environments and quickly lose their mechanical and physical properties. The proper solution for inhibiting the photooxidation of plastics due to the inevitable exposure to light and oxygen is through the addition of efficient ultraviolet absorbers that are capable of acting as efficient scavengers for light and blocking the formation of free radicals within the polymeric chains. The additives should absorb irradiation light directly and decompose peroxide species. In addition, they should be very compatible with the polymers, not alter the color, be used at a very low concentration, and be safe for the environment if released. Progress was made with the design and use of safe additives to enhance plastic stability and, in particular, polystyrene and polyvinyl chloride. Polyphosphates, Schiff bases, and organometallic complexes containing aromatic moieties showed the potential to be used as ultraviolet absorbers for plastics. The damage on the surface of irradiated plastics in the presence of ultraviolet absorbers is low compared with the blank films.
Since the additives are not linked to plastic through covalent bonds, they can be leached to the surrounding environments. The leakage of these chemicals followed by their degradation poses a danger to both animals and humans. Therefore, future research should be attention to the design, synthesis, and use of safe, non-toxic, and highly stable polymeric additives to suppress the degradation of plastic. Some progress was made, but there is still room for further improvements and modifications.
References
- Yousif, E.; Haddad, R. Photodegradation and photostabilization of polymers, especially polystyrene: Review. SpringerPlus 2013, 2, 398, doi:10.1186/2193-1801-2-398.
- Chamas, A.; Moon, H.; Zheng, J.; Qiu, Y.; Tabassum, T.; Jang, J.H.; Abu-Omar, M.; Scott, S.L.; Suh, S. Degradation rates of plastics in the environment. ACS Sustain. Chem. Eng. 2020, 8, 3494–3511, doi:10.1021/acssuschemeng.9b06635.
- Yaqoob, A.A.; Noor, N.H.M.; Serrà, A.; Mohamad Ibrahim, M.N. Advances and challenges in developing efficient graphene oxide-based ZnO photocatalysts for dye photo-oxidation. Nanomaterials 2020, 10, 932, doi:10.3390/nano10050932.
- The State of Plastics: World Environment Day Outlook 2018. Available online: https://www.unep.org/resources/report/state-plastics-world-environment-day-outlook-2018 (accessed on 5 November 2021).
- Plastics—The Facts 2020. An Analysis of European Plastics Production, Demand and Waste Data. Available online: https://issuu.com/plasticseuropeebook/docs/plastics_the_facts-web-dec2020 (accessed on 2 November 2021).
- Wheeler, R.N., Jr. Poly (vinyl chloride) processes and products. Health Perspect. 1981, 41, 123–128, doi:10.1289/ehp.8141123.
- Schyns, Z.O.G.; Shaver, M.M. Mechanical recycling of packaging plastics: A review. Rapid Commun. 2021, 42, 2000415, doi:10.1002/marc.202000415.
- Singh, N.; Hui, D.; Singh, R.; Ahuja, I.P.S.; Feo, L.; Fraternali, F. Recycling of plastic solid waste: A state of art review and future applications. B. Eng. 2017, 115, 409–422, doi:10.1016/j.compositesb.2016.09.013.
- Gewert, B.; Plassmann, M.M.; MacLeod, M. Pathways for degradation of plastic polymers floating in the marine environment. Sci. Process. Impacts 2015, 17, 1513–152, doi:10.1039/C5EM00207A.
- Matthews, C.; Moran, F.; Jaiswal, A.K. A review on European Union’s strategy for plastics in a circular economy and its impact on food safety. Clean. Prod. 2021, 283, 125263, doi:10.1016/j.jclepro.2020.125263.
- Potaufeux, J.-E.; Odent, J.; Notta-Cuvier, D.; Lauro, F.; Raquez, J.-M. A comprehensive review of the structures and properties of ionic polymeric materials. Chem. 2020, 11, 5914–5936, doi:10.1039/D0PY00770F.
- Moretti, E.; Zinzi, M.; Belloni, E. Polycarbonate panels for buildings: Experimental investigation of thermal and optical performance. Energy Build. 2014, 70, 23–35, doi:10.1016/j.enbuild.2013.11.04.
- Turner, A.; Filella, M. Polyvinyl chloride in consumer and environmental plastics, with a particular focus on metal-based additives. Sci. Process. Impacts 2021, 23, 1376–1384, doi:10.1039/D1EM00213A.
- Shah, A.A.; Hasan, F.; Hameed, A.; Ahmed, S. Biological degradation of plastics: A comprehensive review. Adv. 2008, 26, 246–265, doi:10.1016/j.biotechadv.2007.12.005.
- Hahladakis, J.N.; Velis, C.A.; Weber, R.; Iacovidou, E.; Purnell, P. An overview of chemical additives present in plastics: Migration, release, fate and environmental impact during their use, disposal and recycling. Hazard. Mater. 2018, 344, 179–199, doi:10.1016/j.jhazmat.2017.10.014.
- Umar, K.; Yaqoob, A.A.; Ibrahim, M.N.M.; Parveen, T.; Safian, M.T. Environmental applications of smart polymer composites. Smart Polym. Nanocompos. Biomed. Environ. Appl. 2020, 15, 295–320, doi:10.1016/B978-0-12-819961-9.00008-6.
- Albertsson, A.-C.; Karlsson, S. The three stages in degradation of polymers—Polyethylene as a model substance. Appl. Polym. Sci. 1988, 35, 1289–1302, doi:10.1002/app.1988.070350515.
- Chatge, S.; Yang, Y.; Ahn, J.-H.; Hur, H.-G. Biodegradation of polyethylene: A brief review. Biol. Chem. 2020, 63, 27, doi:10.1186/s13765-020-00511-3.
- Singh, B.; Sharma, N. Mechanistic implications of plastic degradation. Degrad. Stab. 2008, 93, 561–584, doi:10.1016/j.polymdegradstab.2007.11.008.
- Vohlídal, J. Polymer degradation: A short review. Teach. Int. 2020, 3, 213–220, doi:10.1515/cti-2020-0015.
- Gryn’ova, G.; Hodgson, J.L.; Coote, M.L. Revising the mechanism of polymer autooxidation. Biomol. Chem. 2011, 9, 480–490, doi:10.1039/C0OB00596G.
- Webb, H.K.; Arnott, J.; Crawford, R.J.; Ivanova, E.P. Plastic degradation and its environmental implications with special reference to poly (ethylene terephthalate). Polymers 2013, 5, 1–18, doi:10.3390/polym5010001.
- Raquez, J.M.; Bourgeois, A.; Jacobs, H.; Degée, P.; Alexandre, M.; Dubois, P. Oxidative degradations of oxodegradable LDPE enhanced with thermoplastic pea starch: Thermo mechanical properties, morphology, and UV-ageing studies. Appl. Polym. Sci. 2011, 122, 489–496, doi:10.1002/app.34190.
- Zheng, Y.; Yanful, E.K.; Bassi, A.S. A review of plastic waste biodegradation. Rev. Biotechnol. 2005, 25, 243–250, doi:10.1080/07388550500346359.
- Müller, R.-J.; Kleeberg, I.; Deckwer, W.-D. Biodegradation of polyesters containing aromatic constituents. Biotechnol. 2001, 86, 87–95, doi:10.1016/S0168-1656(00)00407-7.
- Andrady, A.L. Microplastics in the marine environment. Pollut. Bull. 2011, 62, 1596–1605, doi:10.1016/j.marpolbul.2011.05.030.
- Coyle, R.; Hardiman, G.; O’Driscoll, K. Microplastics in the marine environment: A review of their sources, distribution processes, uptake and exchange in ecosystems. Case Stud. Therm. Environ. Eng. 2020, 2, 100010, doi:10.1016/j.cscee.2020.100010.
- Paulsson, M.; Parkås, J. Review: Light-induced yellowing of lignocellulosic pulps–mechanism and penetrative methods. BioResources 2012, 7, 5995–6040, doi:10.15376/biores.7.4.5995-6040.
- Jin, C.; Christensen, P.A.; Egerton, T.A.; Lawson, E.J.; White, J.R. Rapid measurement of polymer photo-degradation by FTIR spectrometry of evolved carbon dioxide. Deg. Stab. 2006, 91, 1086–1096, doi:10.1016/j.polymdegradstab.2005.07.011.
- Mu, Z.; Chen, Q.; Zhang, L.; Guan, D.; Li, H. Photodegradation of atmospheric chromophores: Changes in oxidation state and photochemical reactivity. Chem. Phys. 2021, 21, 11581–11591, doi:10.5194/acp-21-11581-2021.
- Wang, C.-N.; Torng, J.-H. Experimental study of the absorption characteristics of some porous fibrous materials. Acoust. 2001, 62, 447–459, doi:10.1016/S0003-682X(00)00043-8.
- Ojeda, T. Freitas, A.; Birck, K.; Dalmolin, E.; Jacques, R.; Bento, F.; Camargo, F. Degradability of linear polyolefins under natural weathering. Degrad. Stab. 2011, 96, 703–707, doi:10.1016/j.polymdegradstab.2010.12.004.
- Sharratt, V.; Hill, C.A.S.; Kint, D.P.R. A study of early colour change due to simulated accelerated sunlight exposure in Scots pine (Pinus sylvestris). Degrad. Stab. 2009, 94, 1589–1594, doi:10.1016/j.polymdegradstab.2009.04.010.
- Bais, A.F.; Mckenzie, R.L. Aucamp, P.J.; Ilyas, M.; Madronich, S.; Tourpali, K. Ozone depletion and climate change: Impacts on UV radiation. Photobiol. Sci. 2015, 14, 19–52, doi:10.1039/c4pp90032d.
- Vitt, R.; Laschewski, G.; Bais, A.F.; Diémoz, H.; Fountoulakis, I.; Siani, A.-M.; Matzarakis, A. UV-Index climatology for Europe based on satellite data. Atmosphere 2020, 11, 727, doi:10.3390/atmos11070727.
- Martins, J.N.; Freire, E.; Hemadipou, H. Applications and market of PVC for piping industry. Polímeros 2009, 19, 58–62, doi:10.1590/S0104-14282009000100014.
- Kumagai, H.; Tashiro, T.; Kobayashi, T. Formation of conjugated carbon bonds on poly (vinyl chloride) films by microwave-discharge oxygen-plasma treatments. Appl. Polym. Sci. 2005, 96, 589–594, doi:10.1002/app.21487.
- Yu, J.; Sun, L.; Ma, C.; Qiao, Y.; Yao, H. Thermal degradation of PVC: A review. Waste Manag. 2016, 48, 300–314, doi:10.1016/j.wasman.2015.11.041.
- Miskolczi, N.; Bartha, L.; Angyal, A. Pyrolysis of polyvinyl chloride (PVC)-containing mixed plastic wastes for recovery of hydrocarbons. Energy Fuels 2009, 23, 2743–2749, doi:10.1021/ef8011245.
- Braun, D. Recycling of PVC. Polym. Sci. 2002, 27, 2171–2195, doi:10.1016/S0079-6700(02)00036-9.
- Larché, J.-F.; Bussière, P.-O.; Thérias, S.; Gardette, J.-L. Photooxidation of polymers: Relating material properties to chemical changes. Degrad. Stab. 2012, 97, 25–35, doi:10.1016/j.polymdegradstab.2011.10.020.
- Geuskens, G.; Baeyens-Volant, D.; Delaunois, G.; Lu Vinh, Q.; Piret, W.; David, C. Photo-oxidation of polymers–II. The sensitized decomposition of hydroperoxides as the main path for initiation of the photo-oxidation of polystyrene irradiated at 253.7 nm. Polym. J. 1978, 14, 299–303, doi:10.1016/0014-3057(78)90052-6.
- Yaqoob, A.A.; Noor, N.H.M.; Umar, K.; Adnan, R.; Ibrahim, M.N.M.; Rashid, M. Graphene oxide–ZnO nanocomposite: An efficient visible light photocatalyst for degradation of rhodamine B. Nanosci. 2021, 11, 1291–1302, doi:10.1007/s13204-020-01665-8.
- Huang, Z.; Ding, A.; Guo, H.; Lu, G.; Huang, X. Construction of nontoxic polymeric UV-absorber with great resistance to UV-photoaging. Rep. 2016, 6, 25508, doi:10.1038/srep25508.
- Sonnenschein, M.F.; Guillaudeu, S.J.; Landes, B.G.; Wendt, B.L. Comparison of adipate and succinate polymers in thermoplastic polyurethanes. Polymer 2010, 51, 3685–3692, doi:10.1016/j.polymer.2010.06.012.
- Lu, T.; Solis-Ramos, E.; Yi, Y.; Kumosa, M. UV degradation model for polymers and polymer matrix composites. Degrad. Stab. 2018, 154, 203–210, doi:10.1016/j.polymdegradstab.2018.06.004.
- Rabek, J. Polymer Photodegradation: Mechanisms and Experimental Methods; Champan & Hall: London, UK, 1995; pp. 383–391.
- George, G.A. The mechanism of photoprotection of polystyrene film by some ultraviolet absorbers. Appl. Polym. Sci. 1974, 18, 117–124, doi:10.1002/app.1974.070180110.
- Liu, X.; Gao, C.; Sangwan, P.; Yu, L.; Tong, Z. Accelerating the degradation of polyolefins through additives and blending. Appl. Polym. Sci. 2014, 131, 40750, doi:10.1002/app.40750.
- Balakit, A.A.; Ahmed, A.; El-Hiti, G.A.; Smith, K.; Yousif, E. Synthesis of new thiophene derivatives and their use as photostabilizers for rigid poly (vinyl chloride). J. Polym. Sci. 2015, 2015, 510390, doi:10.1155/2015/510390.
- Yousif, E.; El-Hiti, G.A.; Haddad, R.; Balakit, A.A. Photochemical stability and photostabilizng efficiency of poly (methyl methacrylate) based on 2-(6-methoxynaphthalen-2-yl) propanoate metal ions complexes. Polymers 2015, 7, 1005–1019, doi:3390/polym7061005.
- Yousif, E.; El-Hiti, G.A.; Hussain, Z.; Altaie, A. Viscoelastic, spectroscopic and microscopic study of the photo irradiation effect on the stability of PVC in presence of sulfamethoxazole Schiff’s bases. Polymers 2015, 7, 2190–2204, doi:3390/polym7111508.
- Yousif, E.; Hasan, A.; El-Hiti, G.A. Spectroscopic, physical and topography of photochemical process of PVC films in the presence of Schiff base metal complexes. Polymers 2016, 8, 204, doi:10.3390/polym8060204.
- Ali, M.M.; El-Hiti, G.A.; Yousif, E. Photostabilizing efficiency of poly (vinyl chloride) in the presence of organotin (IV) complexes as photostabilizers. Molecules 2016, 21, 1151, doi:10.3390/molecules21091151.
- Ali, G.Q.; El-Hiti, G.A.; Tomi, I.H.R.; Haddad, R.; Al-Qaisi, A.J.; Yousif, E. Photostability and performance of polystyrene films containing 1,2,4-triazole-3-thiol ring system Schiff bases. Molecules 2016, 21, 1699, doi:10.3390/molecules21121699.
- Mohammed, R.; El-Hiti, G.A.; Ahmed, A.; Yousif, E. Poly (vinyl chloride) doped by 2-(4-isobutylphenyl) propanoate metal complexes: Enhanced resistance to UV irradiation. J. Sci. Eng. 2017, 42, 4307–4315, doi:10.1007/s13369-016-2323-z.
- Ahmed, D.S.; El-Hiti, G.A.; Hameed, A.S.; Yousif, E.; Ahmed, A. New tetra-Schiff bases as efficient photostabilizers for poly (vinyl chloride). Molecules 2017, 22, 1506, doi:3390/molecules22091506.
- Ali, M.M.; El-Hiti, G.A.; Yousif, E. Investigation of the photodecomposition rate constant of poly (vinyl chloride) films containing organotin (IV) complexes. Al-Nahrain J. Sci. 2017, 20, 18–23. doi:10.22401/JUNS.20.3.04.
- Ahmed, D.S.; El-Hiti, G.A.; Yousif, E.; Hameed, A.S. Polyphosphates as inhibitors for poly (vinyl chloride) photodegradation. Molecules 2017, 22, 1849, doi:10.3390/molecules22111849.
- Yousif, E.; Haddad, R.; El-Hiti, G.A.; Yusop, R.M. Spectroscopic and photochemical stability of polystyrene films in the presence of metal complexes. Taibah Univ. Sci. 2017, 11, 997–1007, doi:10.1016/j.jtusci.2017.03.002.
- Ghazi, D.; El-Hiti, G.A.; Yousif, E.; Ahmed, D.S.; Alotaibi, M.H. The effect of ultraviolet irradiation on the physicochemical properties of poly (vinyl chloride) films containing organotin (IV) complexes as photostabilizers. Molecules 2018, 23, 254, doi:10.3390/molecules23020254.
- Shaalan, N.; Laftah, N.; El-Hiti, G.A.; Alotaibi, M.H.; Muslih, R.; Ahmed, D.S.; Yousif, E. Poly (vinyl chloride) photostabilization in the presence of Schiff bases containing a thiadiazole moiety. Molecules 2018, 23, 913, doi:10.3390/molecules23040913.
- Hashim, H.; El-Hiti, G.A.; Alotaibi, M.H.; Ahmed, D.S.; Yousif, E. Fabrication of ordered honeycomb porous poly (vinyl chloride) thin film doped with a Schiff base and nickel (II) chloride. Heliyon 2018, 4, e00743, doi:10.1016/j.heliyon.2018.e00743.
- Yousif, E.; Ahmed, D.S.; El-Hiti, G.A.; Alotaibi, M.H.; Hashim, H.; Hameed, A.S.; Ahmed, A. Fabrication of novel ball-like polystyrene films containing Schiff bases microspheres as photostabilizers. Polymers 2018, 10, 1185, doi:10.3390/polym10111185.
- Alotaibi, M.H.; El-Hiti, G.A.; Hashim, H.; Hameed, A.S.; Ahmed, D.S.; Yousif, E. SEM analysis of the tunable honeycomb structure of irradiated poly (vinyl chloride) films doped with polyphosphate. Heliyon 2018, 4, e01013, doi:10.1016/j.heliyon.2018.e01013.
- El-Hiti, G.A.; Alotaibi, M.H.; Ahmed, A.A.; Hamad, B.A.; Ahmed, D.S.; Ahmed, A.; Hashim, H.; Yousif, E. The morphology and performance of poly (vinyl chloride) containing melamine Schiff bases against ultraviolet light. Molecules 2019, 24, 803, doi:10.3390/molecules24040803.
- Alotaibi, M.H.; El-Hiti, G.A.; Yousif, E.; Ahmed, D.S.; Hashim, H.; Hameed, A.S.; Ahmed, A. Evaluation of the use of polyphosphates as photostabilizers and in the formation of ball-like polystyrene materials. Polym. Res. 2019, 26, 161, doi:10.1007/s10965-019-1829-y.
- Hadi, A.G.; Yousif, E.; El-Hiti, G.A.; Ahmed, D.S.; Jawad, K.; Alotaibi, M.H.; Hashim, H. Long-term effect of ultraviolet irradiation on poly (vinyl chloride) films containing naproxen diorganotin (IV) complexes. Molecules 2019, 24, 2396, doi:10.3390/molecules24132396.
- Hadi, A.G.; Jawad, K.; El-Hiti, G.A.; Alotaibi, M.H.; Ahmed, A.A.; Ahmed, D.S.; Yousif, E. Photostabilization of poly (vinyl chloride) by organotin (IV) compounds against photodegradation. Molecules 2019, 24, 3557, doi:10.3390/molecules24193557.
- Ahmed, A.A.; Ahmed, D.S.; El-Hiti, G.A.; Alotaibi, M.H.; Hashim, H.; Yousif, E. SEM morphological analysis of irradiated polystyrene film doped by a Schiff base containing a 1,2,4-triazole ring system. Petrochem. Res. 2019, 9, 169–177, doi:10.1007/s13203-019-00235-6.
- El-Hiti, G.A.; Ahmed, D.S.; Yousif, E.; Alotaibi, M.H.; Star, H.A.; Ahmed, A.A. Influence of polyphosphates on the physicochemical properties of poly (vinyl chloride) after irradiation with ultraviolet light. Polymers 2020, 12, 193, doi:10.3390/polym12010193.
- Mohammed, A.; El-Hiti, G.A.; Yousif, E.; Ahmed, A.A.; Ahmed, D.S.; Alotaibi, M.H. Protection of poly (vinyl chloride) films against photodegradation using various valsartan tin complexes. Polymers 2020, 12, 969, doi:10.3390/polym12040969.
- Ahmed, D.S.; El-Hiti, G.A.; Ibraheem, H.; Alotaibi, M.H.; Abdallh, M.; Ahmed, A.A.; Ismael, M.; Yousif, E. Enhancement of photostabilization of poly (vinyl chloride) doped with sulfadiazine tin complexes. Vinyl Addit. Techn. 2020, 26, 370–379, doi:10.1002/vnl.21752.
- Mahmood, Z.N.; Yousif, E.; Alias, M.; El-Hiti, G.A.; Ahmed, D.S. Synthesis, characterization, properties, and use of new fusidate organotin complexes as additives to inhibit poly (vinyl chloride) photodegradation. Polym. Res. 2020, 27, 267, doi:10.1007/s10965-020-02245-8.
- Majeed, A.; Yousif, E.; El-Hiti, G.A.; Alotaibi, M.H.; Ahmed, D.S.; Ahmed, A.A. Stabilization of PVC containing captopril tin complexes against degradation upon exposure to ultraviolet light. Vinyl Addit. Techn. 2020, 26, 601–612, doi:10.1002/vnl.21774.
- Salam, B.; El-Hiti, G.A.; Bufaroosha, M.; Ahmed, D.S.; Ahmed, A.; Alotaibi, M.H.; Yousif, E. Tin complexes containing an atenolol moiety as photostabilizers for poly (vinyl chloride). Polymers 2020, 12, 2923, doi:10.3390/polym12122923.
- Omer, R.M.; Al-Tikrity, E.T.B.; Yousif, E.; El-Hiti, G.A.; Ahmed, D.S.; Ahmed, A.A. Spectroscopic and morphological study of irradiated PVC films doped with polyphosphates containing 4,4’-methylenedianiline. J. Appl. Chem. 2020, 93, 1888–1898, doi:10.1134/S1070427220120113.
- Mohamed, S.H.; Hameed, A.S.; El-Hiti, G.A.; Ahmed, D.S.; Kadhom, M.; Baashen, M.A.; Bufaroosha, M.; Ahmed, A.A.; Yousif, E. A process for the synthesis and use of highly aromatic organosilanes as additives for poly (vinyl chloride) films. Processes 2021, 9, 91, doi:10.3390/pr9010091.
- Mousa, O.G.; El-Hiti, G.A.; Baashen, M.A.; Bufaroosha, M.; Ahmed, A.; Ahmed, A.A.; Ahmed, D.S.; Yousif, E. Synthesis of carvedilol-organotin complexes and their effects on reducing photodegradation of poly (vinyl chloride). Polymers 2021, 13, 500, doi:10.3390/polym13040500.
- Ahmed, A.; El-Hiti, G.A.; Hadi, A.G.; Ahmed, D.S.; Baashen, M.A.; Hashim, H.; Yousif, E. Photostabilization of poly (vinyl chloride) films blended with organotin complexes of mefenamic acid for outdoor applications. Sci. 2021, 11, 2853, doi:10.3390/app11062853.
- Jasem, H.; Hadi, A.G.; El-Hiti, G.A.; Baashen, M.A.; Hashim, H.; Ahmed, A.A.; Ahmed, D.S.; Yousif, E. Tin-naphthalene sulfonic acid complexes as photostabilizers for poly (vinyl chloride). Molecules 2021, 26, 3629, doi:10.3390/molecules26123629.
- Ghani, H.; Yousif, E.; Ahmed, D.S.; Kariuki, B.M.; El-Hiti, G.A. Tin Complexes of 4-(Benzylideneamino) benzenesulfonamide: Synthesis, structure elucidation and their efficiency as PVC photostabilizers. Polymers 2021, 13, 2434, doi:10.3390/polym13152434.
- Yaseen, A.A.; Al-Tikrity, E.T.B.; Yousif, E.; Ahmed, D.S.; Kariuki, B.M.; El-Hiti, G.A. Effect of ultraviolet irradiation on polystyrene containing cephalexin Schiff bases. Polymers 2021, 13, 2982, doi:10.3390/polym13172982.
- Yaseen, A.A.; Yousif, E.; Al-Tikrity, E.T.B.; El-Hiti, G.A.; Kariuki, B.M.; Ahmed, D.S.; Bufaroosha, M. FTIR, weight, and surface morphology of poly (vinyl chloride) doped with tin complexes containing aromatic and heterocyclic moieties. Polymers 2021, 13, 3264, doi:10.3390/polym13193264.
- Hadi, A.G.; Baqir, S.J.; Ahmed, D.S.; El-Hiti, G.A.; Hashim, H.; Ahmed, A.; Kariuki, B.M.; Yousif, E. Substituted organotin complexes of 4-methoxybenzoic acid for reduction of poly (vinyl chloride) photodegradation. Polymers 2021, 13, 3946, doi:10.3390/polym13223946.