Improvements in the growth, yield, and quality of horticultural crops require the development of simply integrated, cost-efficient, and eco-friendly solutions. Hydrogen gas (H2) has been observed to have fertilization effects on soils by influencing rhizospheric microorganisms, resulting in improvements in crop yield and quality. Ample studies have shown that H2 has positive effects on horticultural crops, such as promoting root development, enhancing tolerance against abiotic and biotic stress, prolonging storage life, and improving postharvest quality of fruits, vegetables and cut flowers.
1. Introduction
Horticultural crops are grown for food, medical use, and aesthetic enjoyment. They form an important part of agricultural production and contribute to food security as well as nutritional quality. The improvement in the growth, yield, and quality of horticultural crops has attracted widespread attention, especially for developing easy, cheap, and eco-friendly solutions, which is a challenge for a low-carbon society.
Hydrogen is the lightest and most abundant chemical element in the universe. Researchers have proposed that hydrogen gas (H
2) played a critical role in the origin of eukaryotes
[1]. Meanwhile, the production and release of H
2 has been observed in algae, animals, and plants
[2][3][4][2,3,4]. Thus, it is not surprising that H
2 has increasingly been attached to various biological functions in animals and plants, which have been observed during the last two decades of studies
[5][6][7][5,6,7].
Despite its low mixing ratio (~530 parts per billion by volume) in current Earth’s atmosphere, H
2 contributes to the homeostasis of the oxidation state in the atmosphere
[8]. In the context of H
2 biogeochemical cycles, the most important source of H
2 for the atmosphere is methane, while other sources are non-methane hydrocarbons and photochemical oxidation. Conversely, microbial-mediated soil uptake is responsible for ~80% of the tropospheric H
2 losses. H
2 has been shown to maintain microbial viability and activity and, in turn, driven carbon cycling
[9]. Since H
2 exposed soil improved plant growth, it has been proposed that H
2 fertilization of soil can be attributed to H
2-oxidizing bacteria in the rhizosphere
[10]. Accordingly, the deliberate application of H
2 might have substantial potential in agricultural benefits.
In 2003, Dong et al.
[10] observed that H
2-treated soil improved growth in canola (
Brassica napus) and first proposed the “H
2 fertilization” hypothesis. Since then, a growing number of studies on the application of H
2 in horticulture have been carried out due to its unique properties in stimulating or sustaining plant growth and development, as well as postharvest preservation in particular (
Figure 1). So far, there are a total of 62 publications on horticultural H
2 application from China (59), Australia (2), and Canada (1). In 2013, H
2 supplied by hydrogen-rich water (HRW) was observed to enhance plant tolerance with respect to herbicide (paraquat), drought, salinity, and cold stress in alfalfa seedlings
[11]. Subsequently, many additional functions of H
2 have been discovered, such as promoting root development in cucumber (
Cucumis sativus)
[12] and tomato (
Lycopersicon esculentum)
[13] and alleviating heavy metal toxicity in pak choi (
Brassica rapa var.
chinensis)
[14] and alfalfa (
Medicago sativa)
[15]. In addition, H
2 has been shown to improve the yield and quality of daylily (
Hemerocallis fulva L.)
[16], as well as prolonging the shelf life and vase life of fruits and flowers including kiwifruit (
Actinidia chinensis var.
deliciosa)
[17], lychee (
Litchi chinensis)
[18], rose (
Rosa chinensis)
[19], and carnation (
Dianthus caryophyllus)
[20] (
Figure 2). As the mechanism underlying the positive effects of H
2 on horticultural crops is progressively being revealed, the values of the application of H
2 in horticulture are being increasingly realized.
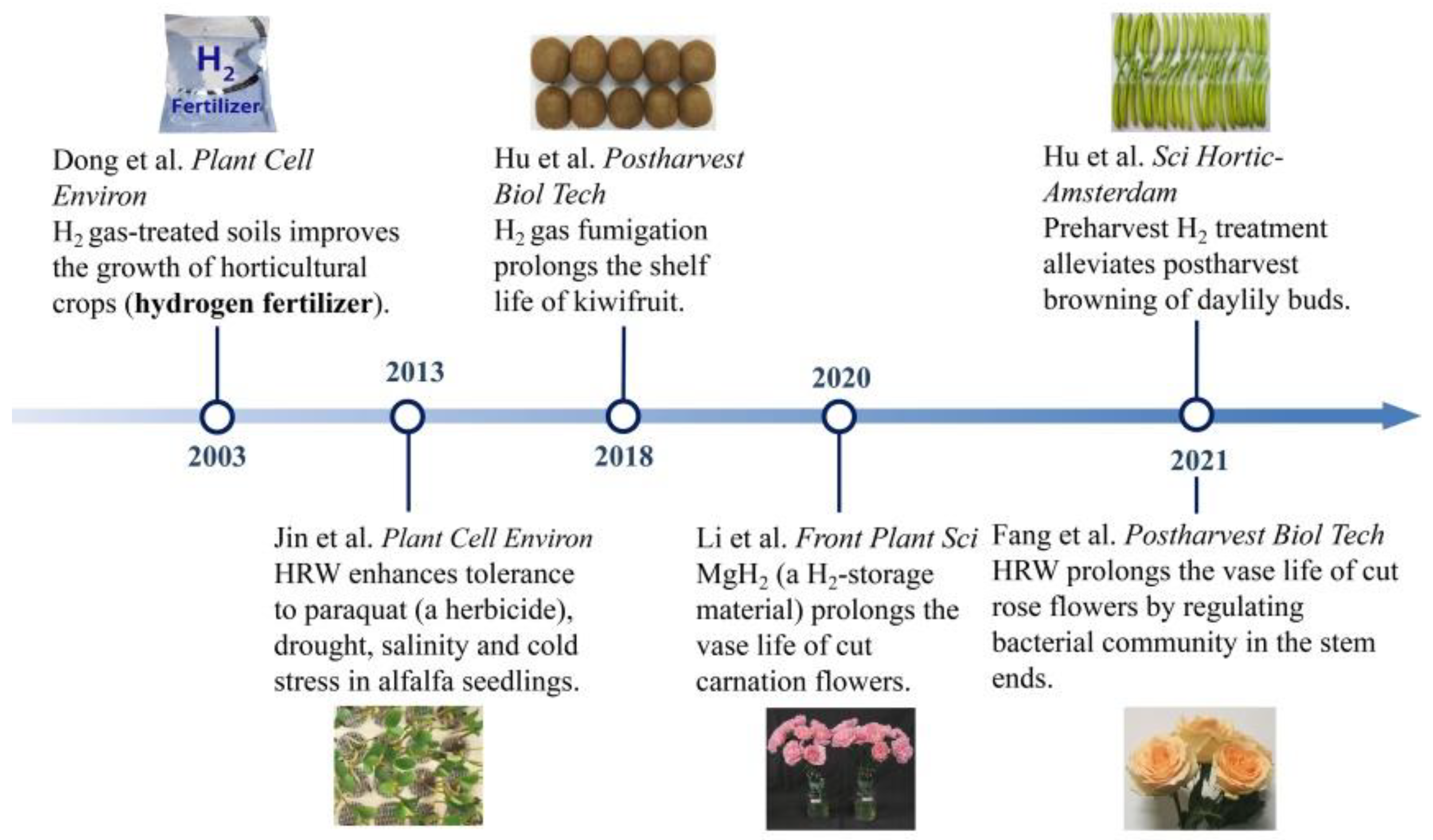
Figure 1. The developing profiles of the application of H2 in horticulture.
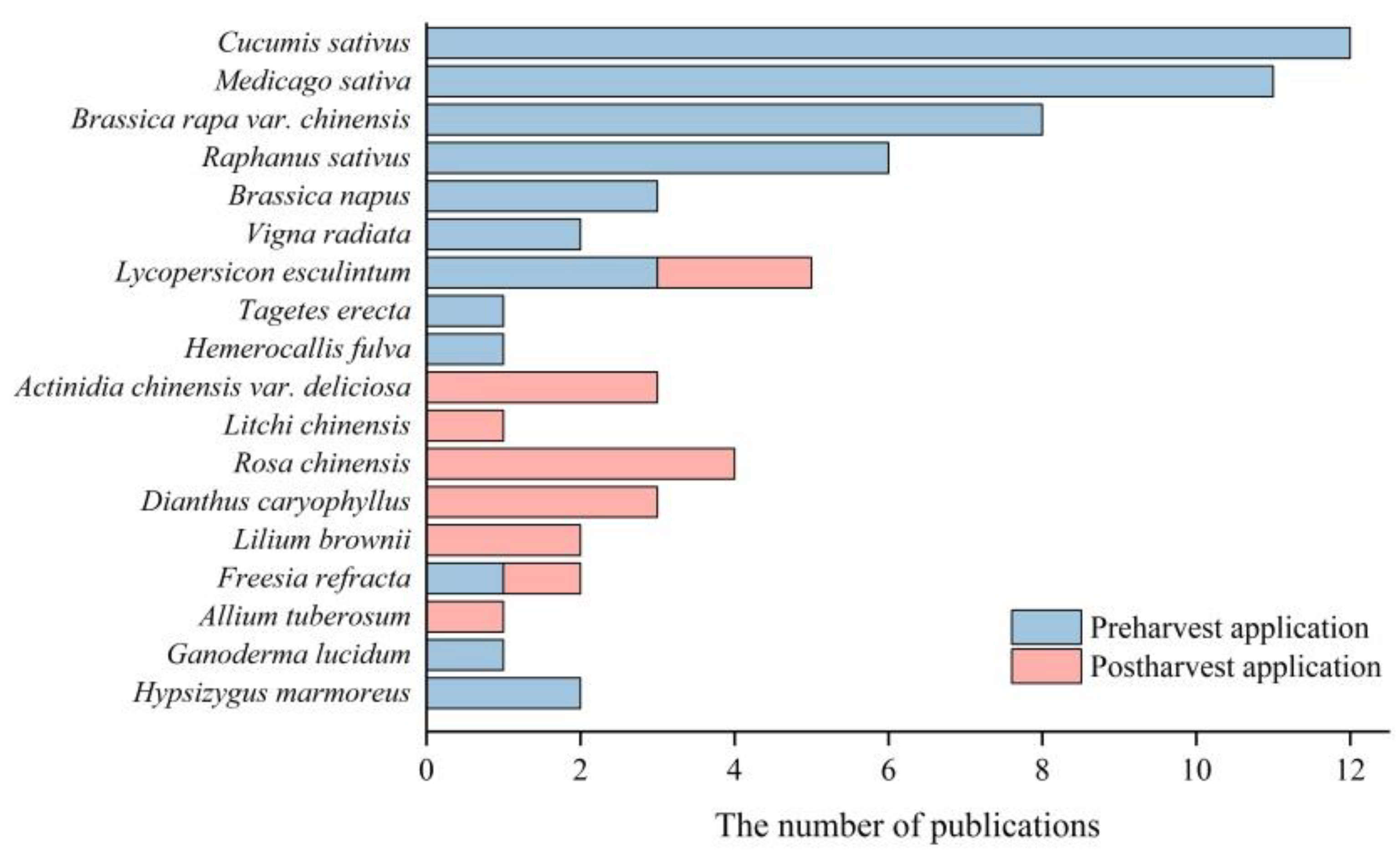
Figure 2. The species of the publications studied on the application of H2 in horticulture.
2. Possible Mechanisms Underlying H2 Responses in Horticultural Crops
2.1. Involved in Reactive Oxygen Species (ROS) and Reactive Nitrogen Species (RNS) Metabolism
Reactive oxygen species (ROS) and reactive nitrogen species (RNS) are commonly involved in plants responses to various stresses
[21][69]. For example, chilling
[22][33], osmatic
[23][24][38,70], paraquat stresses
[11], and metal exposure
[25][26][27][41,53,71] can induce ROS (including superoxide anions (O
2−), hydrogen peroxide (H
2O
2), hydroxyl radical (·OH), etc.) and RNS (nitric oxide (NO), peroxynitrite (ONOO
−), etc.), disturbing the delicate redox homeostasis and causing cellular damage inside the plant cells. In postharvest fruits, vegetables, and cut flowers, ROS overproduction accelerated senescence process
[16][17][18][28][29][16,17,18,21,52]. Additionally, ROS and RNS are vital signaling transducers in plant signaling networks for stress and development
[30][72]. Therefore, the metabolic regulation of ROS and RNS is crucial for stress responses, growth, and development in plants.
Endogenous H
2 could be produced under abiotic stresses and senescence conditions in plants
[11][31][32][29][11,32,34,52]. H
2 can increase the activities of antioxidant enzymes such as superoxide dismutase (SOD), catalase (CAT), guaiacol peroxidase (POD), and ascorbate peroxidase (APX) and the transcript levels of corresponding genes, thus resulting in scavenging overproduced ROS and reestablishing redox homeostasis in alfalfa seedlings subjected to osmotic stress
[11][24][11,70] (
Table 1).
Table 1. Role of H2 involved in reactive oxygen species (ROS) and reactive nitrogen species (RNS) metabolism in horticultural crops.
Materials |
Treatment Stage |
H | 2 | Delivery Methods and Treatment |
Effective Concentration of H | 2 |
Functions of H | 2 |
Mechanism |
Ref. No. |
Brassica rapa var. chinensis | ‘Dongfang 2′ |
Preharvest |
1/4 Hoagland’s nutrient solution with H | 2 | (830 µM); the seedlings were pretreated for 48 h |
~415 µM |
Alleviates cadmium toxicity |
Regulates NR-dependent NO signaling and enhances antioxidant capacity |
[26] | [53] |
1/4 Hoagland solution with H | 2 | (865 µM); the seedlings were pretreated for 2/3 d (replaced every 12 h) |
865 µM |
Reduces cadmium uptake in plant roots |
Control of NADPH oxidase encoded by | RbohD | , which operates upstream of IRT1, and regulates root Cd uptake at both the transcriptional and functional levels |
[33] | [54] |
Medicago sativa | ‘Biaogan’ |
Preharvest |
HRW (220 µM); the seedlings were pretreated for 12 h |
~110 µM |
Enhances tolerance to paraquat |
Modulates HO-1 signaling |
[11] |
Alleviates aluminum toxicity |
Decreases NO production |
[25] | [41] |
HRW (780 µM); the seedlings were pretreated for 12 h |
~390 µM |
Induces osmotic stress tolerance |
Regulates H | 2 | O | 2 | and HO-1 signaling |
[23] | [38] |
NO-mediated proline accumulation and reestablishment of redox balance |
[24] | [70] |
Cucumis sativus | ‘Xinchun 4′ |
Preharvest |
HRW (450 µM);
the seedlings were incubated for 2/5 d (changed daily) |
~225 µM |
Promotes adventitious rooting |
Regulates CO signaling and activates antioxidant system |
[32] | [34] |
Regulates NO signaling |
[34][35] | [73,74] |
Induces adventitious rooting under cadmium stress |
Decreases oxidative damage, increases osmotic adjustment substance content, and regulates rooting-related enzyme activity |
[27] | [71] |
Cucumis sativus | ‘Jinyou 35′ |
Preharvest |
HRW (450 µM); the seeds were soaked for 8 h |
450 µM |
Enhances cold tolerance |
Enhances antioxidant capacity and slows dehydration rate by improving osmotic adjustment ability |
[22] | [33] |
Solanum lycopersicum | ‘Baiguoqiangfeng’ |
Preharvest |
AB@hMSN (10 mg/L); the seedlings were incubated for 2/5 d |
~400 µM |
Induces lateral root formation |
Modulates NR-dependent NO synthesis, cell cycle regulatory genes, and miRNAs expression |
[36] | [30] |
Hypsizygus marmoreus |
Preharvest |
HRW (1000 µM); the mycelia were cultivated until harvesting |
~250 µM |
Increases postharvest quality |
Enhances antioxidant defense |
[37] | [46] |
Hemerocallis fulva | ‘Dawuzui’ |
Preharvest |
HRW (1.6 µM);
irrigation at the stages of bolting, growing and the day prior to the period of harvest |
~0.8 µM |
Promotes daylily bud yield and alleviation of bud browning |
Decreases ROS level, increases the unsaturated:saturated fatty acid ratio, endogenous H | 2 | and total phenol content, and reduces PAL and PPO activity |
[16] |
Actinidia chinesis | ‘Huayou’ |
Postharvest |
HRW (660 µM); the fruits were soaked for 5 min |
~528 µM |
Delays postharvest ripening and senescence |
Enhances antioxidant defense |
[17] |
Litchi chinensis | ‘Huaizhi’ |
Postharvest |
HRW (500 µM); the fruits were soaked for 3 min |
~350 µM |
Delays the pericarp browning |
Induces antioxidant system-related characters |
[18] |
Rosa chinensis | ‘Kardinal’; | Lilium brownii | ‘Manissa’ |
Postharvest |
HRW (450 µM); cut flowers were incubated for vase period (changed daily) |
~225 µM (Rose);
~45 µM (Lily) |
Improves the vase life and quality |
Maintains water balance and membrane stability by reducing stomatal size and oxidative damage |
[19] |
Allium tuberosum |
Postharvest |
Gas; the leaves were fumigated for storage period (renewed daily) |
~1.2×10 | 3 | µM |
Prolongs the shelf life and maintain storage quality |
Increases antioxidant capacity |
[28] | [21] |
Dianthus caryophyllus | ‘Pink Diamond’ |
Postharvest |
HNW (~500 µM); cut flowers were incubated for 3 d (changed daily) |
~50 µM |
Prolongs the vase life |
Reduces ROS accumulation and senescence-associated enzyme activities |
[38] | [26] |
Rosa chinensis | ‘Carola’ |
Postharvest |
MgH | 2 | (0.001 g/L); cut flowers were incubated for vase periods (changed daily) |
Not shown |
Prolongs the vase life |
Maintains ROS balance by modulating NO synthesis |
[39] | [28] |
Lilium brownii | ‘Manissa’ |
Postharvest |
HRW; cut flowers were incubated for vase period (changed daily) |
Not shown (1% saturation HRW) |
Prolongs the vase life |
Regulates NO signaling and regulates the expression of the photosynthesis-related AtpA |
[40] | [50] |
Freesia refracta | ‘Red passion’ |
Postharvest |
HRW (75 µM); cut flowers were pretreated for 12 h |
~0.75 µM |
Prolongs the vase life |
Improves antioxidant capacity |
[41] | [51] |
Eustoma grandiflorum |
Postharvest |
HRW (780 µM); cut flowers were incubated for vase period (changed daily) |
~78 µM |
Prolongs the vase life |
Maintains redox homeostasis |
[29] | [52] |
2.2. Modulation in Sulfur Compounds’ Metabolism
Sulfur assimilation, cysteine and methionine metabolism, and GSH metabolism eventually influence plant growth, development, and stress responses
[42][75]. For example, under Cd stress, HRW upregulated the genes involved in sulfate absorption, transport, and sulfur assimilation (including
ATP sulfurylases, 5′-adenylylsulfate reductases,
O-acetylserine(thiol)lyase,
glutathione S-transferase (GST),
cysteine desulfurases, etc.), thus increasing sulfur contents of both leaves and roots in alfalfa
[43][44][76,77] (
Table 2). GSH content and GSH/GSSG ratio increased after HRW pretreatment by increasing the transcripts of
glutathione synthase (
GS) and
glutathione reductase (
GR)
[15][45][46][15,39,40], as well as phytochelatins (PCs) content
[43][76], thus associating with Cd chelation and antioxidant capacity in pak choi. Subsequently, H
2 was observed to increase transcript levels of
SlGSH1 and
SlGSH2 that encode
γ-glutamylcysteine synthetase (
γ-ECS) and GS, confirming the stimulation of GSH synthesis and, thus, resulting in inducing lateral root branching of tomato
[47][35].
Table 2. Role of H
2 involved in sulfur compounds metabolism in horticultural crops.
Materials |
Treatment Stage |
H | 2 | Delivery Methods and Treatment |
Effective Concentration of H | 2 |
Functions of H | 2 |
Mechanism |
Ref. No. |
Brassica rapa | var. | chinensis | ‘Dongfang 2′ |
Preharvest |
1/4 Hoagland’s nutrient solution with H | 2 | ; the seedlings were incubated for 48 h (replaced every 12 h) after removing cadmium stress |
Not shown (50% saturation HRW) |
Enhances cadmium tolerance |
Reestablishes reduced GSH homeostasis |
[45] | [39] |
Medicago sativa | ‘Victoria’ |
Preharvest |
HRW (220 µM); the seedlings were pretreated for 12 h |
~22 µM |
Alleviates cadmium toxicity |
Reduces cadmium accumulation and reestablishes GSH homeostasis |
[15] |
Expression regulation of genes relevant to sulfur and glutathione metabolism, resulting in enhanced glutathione metabolism and activating antioxidant defense and cadmium chelation |
[43] | [76] |
Decreases oxidative damage, enhances sulfur compound metabolic process, and reestablishes nutrient element homeostasis |
[44] | [77] |
Alleviates mercury toxicity |
Reduces mercury accumulation and reestablishes redox homeostasis (GSH, AsA, and antioxidant enzymes) |
[46] | [40] |
Solanum lycopersicum | ‘Baiguoqiangfeng’ |
Preharvest |
HRW (780 µM); the seedlings were incubated for 4 d (changed daily) |
~390 µM |
Influences lateral root branching |
Promotes γ-ECS-dependent GSH production |
[47] | [35] |
Ganoderma lucidum | strain HG |
Preharvest |
HRW (220 µM); added to the medium after 4 days of mycelium culture. |
~11 µM |
Regulates morphology, growth, and secondary metabolism |
Increases glutathione peroxidase activity under HAc stress |
[48] | [42] |
Dianthus caryophyllus | ‘Pink Diamond’ |
Postharvest |
MgH | 2 | (0.1 g/L MgH | 2 | and 0.1 M PBS (pH 3.4); cut flowers were incubated for vase period (changed daily) |
~400 µM |
Prolongs the vase life |
H | 2 | S-mediated reestablishment of redox homeostasis and increased transcript levels of | DcbGal | and | DcGST1 |
[49] | [25] |
2.3. Involvement in Flavonoids Metabolism
In plants, flavonoids and their glycoconjugates (glycosides) have evolved to protect against ultraviolet radiation (UV)-triggered oxidative damage
[50][82]. Xie et al.
[51][83] found that under UV-B irradiation, HRW promoted alfalfa tolerance to UV-B stress, accompanied by enhancement of flavonoids profiles (included isoflavone, flavanone, flavonol, chalcone, and pterocarpan). HRW can increase transcript levels of flavonoids biosyntheticrelated genes, including
L-phenylalanin ammonialyase (
PAL),
chalcone synthase (
CHS),
chalcone isomerase (
CHI),
flavonol synthase (
FLS),
isoflavone synthase (
IFS), and
isoflavone 6-O-methyl transferase (
6IOMT) (
Table 3).
Table 3. Role of H2 involved in flavonoids metabolism in horticultural crops.
Materials |
Treatment Stage |
H | 2 | Delivery Methods and Treatment |
Effective Concentration of H | 2 |
Functions of H | 2 |
Mechanism |
Ref. No. |
Raphanus sativus | ‘Qingtou’;
| R. sativus | ‘Yanghua’ |
Preharvest |
HRW (220 µM); 1/4 Hoagland’s nutrient solution with H | 2 | (220 µM H | 2 | ); the seeds were soaked in HRW for 12 h; sprouts were incubated in nutrient solution with H | 2 | for 3 d (replaced every 12 h) under UV-A |
~220 µM |
29][52], and H
2 could, in turn, have an impact on photosynthesis
[31][32] (
Table 4).
Table 4. Roles of H2 involved in carbon and nitrogen metabolism in horticultural crops.
Materials |
Treatment Stage |
H | 2 | Delivery Methods and Treatment |
Effective Concentration of H | 2 |
Functions of H | 2 |
Mechanism |
Ref. No. |
Regulates anthocyanin synthesis under UV-A |
Cucumis sativus | ‘XinJinchun No. 4′ |
Preharvest |
Hoagland’s nutrient solution with H | 2 | (220 µM H | 2 | ); the seedlings were pretreated for 7 d (replaced daily)Reestablishes ROS homeostasis and regulates anthocyanin biosynthesis-related gene expression |
[52] | [37] |
~110 µM |
Improves heat tolerance |
Improves photosynthetic and antioxidant and increases HSP70 content |
[ | 31 | ] | [32] |
~585 µM |
Reduces nitrite accumulation during storage |
Inhibits/increases the activity and transcript level of NR/NiR |
[ | 59 | ] | [ | 47] |
2.5. Modulation of Ion Homeostasis
Ion homeostasis plays an important role in plant tolerance to drought, salinity, and heavy metal stress
[60][93]. It has been observed that NH
3∙BH
3 can decrease Na content and increase K content, resulting in a decreased Na/K ratio in rapeseed seedling roots subjected to NaCl stress
[61][29] (
Table 5).
Table 5. Roles of H2 involved in modulation of ion homeostasis in horticultural crops.
Materials |
Treatment Stage |
H | 2 | Delivery Methods and Treatment |
Effective Concentration of H | 2 |
Functions of H | 2 |
Mechanism |
Ref. No. |
Brassica rapa | var. | chinensis | ‘Dongfang 2’ |
Preharvest |
1/4 Hoagland’s nutrient solution with H | 2 | ; the seedlings were pretreated for 1 d (replaced every 12 h) |
Not shown (50% saturation HRW) |
Reduces cadmium accumulation |
Inhibits the expression of | BcIRT1 | and | BcZIP2 | , and reduces cadmium absorption |
[62][63] | [94,95] |
Raphanus. sativus |
Brassica rapa | var. | chinensis | ‘Dongfang 2′ |
Preharvest |
Brassica napus | ‘Zhongshuang 11′ | HRW; 1/4 Hoagland’s nutrient solution with H2 (835.1 μM H | 2 |
Preharvest); regarding soil cultivation, sprays with HRW (50 mL) at every 12 h for 17 d; for hydroponic solutions, the seedlings were incubated in 1/4 Hoagland solution with H | 2 | for 4 d (replaced every 12 h) with Ca(NO | 3 | ) | 2 |
Ammonia borane (NH | 3 | ∙BH~417 µM |
2.6. H2 Is Involved in Phytohormones Signaling
Abscisic acid (ABA), ethylene (ETH), and jasmonate acid (JA) can induce H
2, but the specific biosynthesis pathway has yet to be elucidated
[65][66][31,97]. For alfalfa drought response, H
2 acted as a positive regulator in the ABA signaling cascade to regulate stomatal movement
[66][97] (
Table 6). H
2-modified apoplastic pH by H
+-ATPase might be involved in this signaling process. Moreover, H
2 differentially increased the transcriptional factor genes involved in ABA signaling, including
MYB102,
MYC2, and
ABF/
AREB2 [67][98].
Table 6. Roles of H2 involved in phytohormones signaling in horticultural crops.
Materials |
Treatment Stage |
H | 2 | Delivery Methods and Treatment |
Effective Concentration of H | 2 |
Functions of H | 2 |
Mechanism |
Ref. No. |
Medicagosativa | ‘Victoria’ |
Preharvest |
HRW; the seedlings were irrigated for 7 d before 15-d drought treatment |
Not shown (50% saturation HRW) |
Induces drought tolerance |
Modulates stomatal sensitivity to ABA and Apoplastic pH |
[66] | [97] |
‘Yanghua’ |
Preharvest |
HRW (781 µM); the seedlings were incubated for 48/60 h (replaced every 12 h) under UV-A |
3 | ~781 µM |
; 2 mg/L); the seedlings were incubated for 3 d (changed daily) under NaCl, PEG, or Cd stress | Promotes the biosynthesis of anthocyanin under UV-A |
Reduces Ca(NO | 3 | Regulates InsP | 3 | -dependent calcium signaling |
) | [53] |
Medicagosativa | ‘Victoria’ | ~300 µM | 2 | [ | 86 | ] |
toxicity and improves the growth of seedlings |
Enhances antioxidant capacities and reestablishes nitrate homeostasis |
[ |
Enhances the tolerance against salinity, drought, or cadmium | 56 |
Preharvest |
1/4 Hoagland’s nutrient solution with H | 2 | (780 µM H | 2 | ); the seedlings were pretreated for 12 hDecreases cell death rebuilds redox and ion homeostasis, increases proline content, thus reducing cadmium absorption and accumulation | ] | [44] |
[ | 61 | ] | [ | 29 | ] |
~390 µM |
Induces tolerance against osmotic stress |
Involved in phytohormone signaling |
[67] | [98] |
Involved in phytohormones, MAPKs and Ca | 2+ | signaling |
Cucumis sativus | ’Jinyou 35′ |
Preharvest |
HRW (450 µM); the seeds were soaked for 8 h |
Cucumis sativus | ~450 µM |
‘Xinchun 4′ | [54] | [87] |
Enhances lower temperature tolerance |
Increases the activities of key photosynthetic enzymes and maintains a high level of carbon and nitrogen metabolism |
Preharvest | [ | 57 | ] | [90] |
Cucumis sativus | ‘Xinchun 4′ |
Preharvest |
HRW (450 µM); the seedlings incubated for 2/5 d (changed daily) |
~450 µM |
HRW (680 µM); the seedlings were incubated for 7 d (changed daily) | Induces adventitious rooting |
~350 µM | Regulates the protein and gene expressions of PM H | + | -ATPase and 14-3-3 mediated by NO. |
Induces adventitious rooting | [64 |
Ethylene may be the downstream signaling molecule during H | 2 | ] | [96] |
-induced adventitious rooting, and proteins RuBisCo, SBPase, OEE1, TDH, CAPX, and PDI may play important roles |
[68] | [100] |
HRW (220 µM); the seedlings were incubated for 72 h (replaced every 12 h) under short wavelength light |
Hypsizygus marmoreus | ~220 µM |
PreharvestPromotes anthocyanin accumulation under short wavelength light |
HRW (800 µM); mycelia were incubated for 5 d (replaced every 12 h) after removal of cadmium stressPromotes activities and transcription of anthocyanin biosynthesis-related enzyme (including CHS and UFGT) |
~800 µM |
Alleviates salinity and heavy metal toxicity |
Activates pyruvate kinase, along with its induced gene expression |
[58] |
Cucumis sativus | ‘Lufeng’ | [ | 55 | ] | [88] |
[ |
Preharvest |
HRW (220 µM); incubated for 4 d |
~110 µM |
Regulates adventitious root development |
Regulates HO-1 signaling | 43] |
[ | 12 | ] |
Medicagosativa | ‘Victoria’ |
Preharvest |
Solanum lycopersicum | ‘Jiafen No. 2′ |
Vigna radiata | ; | Cucumis sativus | ‘Jinchun 4′; | Raphanus sativus | HRW (781 µM); the seedlings were pretreated for 12 h |
Postharvest |
‘Yanghua’ |
Preharvest | HRW (780 µM); the fruits were soaked for 20 min~390 µM |
1/8 strength Hoagland nutrition solution with H |
2 |
(800 µM); the seedlings were incubated for 5 d (replaced every 12 h) |
Alleviates UV-B-triggered oxidative damage |
Regulates (iso)flavonoids metabolism and antioxidant defense |
[ | 51 | ] | [ | 83] |
2.4. H2 Is Involved in Carbon and Nitrogen Metabolism
A previous study has observed that endogenous H
2 production can be inhibited by an inhibitor of photosynthetic electron flow, indicating that, in plants, endogenous H
2 production may be associated with photosynthesis
[
~480 µM |
Promotes elongation of hypocotyls and roots |
Increases GA and IAA contents in the hypocotyl and the root |
[ | 69 | ] | [ | 99 | ] |
Vigna radiata |
Preharvest |
HRW; seeds were soaked for 3 d |
100/250 µM |
Promotes the growth of shoots and roots |
Involved in phytohormone signaling |
[65] | [31] |
Freesia refracta |
Preharvest |
HRW (75 µM); the bulbs were soaked for 6 h; irrigated HRW at every 7–10 d and total 3 times after scape sticking out |
~37.5 µM |
Promotes early flowering; increases the number and diameters of florets |
Regulates phytohormone and soluble sugar content |
[70] | [45] |
Actinidia deliciosa | ‘Xuxiang’ |
Postharvest |
Gas; the fruits were fumigated for 24 h/12 h + 12 h |
~0.2 µM |
Prolongs the shelf life |
Decreases ethylene biosynthesis |
[71] | [22] |
Rosa chinensis | ‘Movie star’ |
Postharvest |
HRW (235 µM); cut flowers were incubated for vase periods (changed daily) |
~2.35 µM |
Alleviates postharvest senescence |
Inhibits ethylene production and alleviates ethylene signal transduction |
[72] | [49] |
3. Conclusions and Prospects
Maintaining or increasing horticultural yield requires NPK fertilizers, manure, hazardous preservatives, or other polluting methods, which could be offset via cleaner or healthier alternatives. H
2 is a carbon-free energy carrier that may be an attractive plant growth regulator for horticultural sustainability. Currently, over 95% of H
2 is made by using fossil fuels, with the most common process of H
2 production being steam methane reformation, which may produce H
2 for ~USD 1.15/kg H
2 in the US
[73][101]. Other H
2 production technologies, such as water electrolysis, are estimated to produce H
2 for ~USD 5.50 per kilogram of H
2. Although renewable H
2 is relatively expensive, its production costs are reducing. According to the BloombergNEF’s report of “Hydrogen Economy Outlook”
[74][102], between 2014 and 2019, the cost of alkaline electrolyzers fell 40% in North America and Europe, and systems made in China are already up to 80% cheaper than those made elsewhere. They forecast that renewable H
2 could be produced for USD 0.7 to USD 1.6/kg H
2 in most parts of the world before 2050. Thus, the cost for applying H
2 in horticulture is primarily dependent on the cost of labor, which is both feasible and affordable, at least under current economic conditions.
H
2 has been applied in the above-mentioned important horticultural crops, confirming its positive effects both on plant growth, development, stress tolerance, and postharvest storage (
Figure 3). A recent field trial has observed that H
2 infusion increased H
2-oxidizing bacteria activities, accompanied with an alteration of composition and structure of the microbial community
[75][103]. However, the above effects of H
2 on soil microbe were significantly influenced by environmental conditions, which would be taken into account in further H
2 field trials. The potential negative effect of H
2 on soil ecosystems should also be concerning. For example, H
2 exposure may stimulate methane oxidation and the activities of pathogens that use H
2 as an energy source
[9]. Therefore, long-term and large-scale commercial field trials of H
2 require further investigation, especially in the evaluation of resistance to pests and diseases, yield, and quality, as well as environmental impact. In addition, enhanced understanding is required with respect to the causal mechanisms underlying plant H
2 production and action.
Overall, H2 has a substantial potential in horticultural applications to reduce fertilizer and pesticide use, providing higher-value and nutrient-rich horticultural crops. Since making technology cheap requires technological advance, we urge the cooperation of the industrial community. The next step may focus on practical application of H2 in horticulture.