The tremendous increase in the production and consumption of titanium dioxide (TiO2) nanoparticles (NPs) in numerous industrial products and applications has augmented the need to understand their role in wastewater treatment technologies. The use of TiO2 NPs as the representative of photocatalytic technology for industrial wastewater treatment is coming to the horizon. As the use of industrial wastewater to feed agriculture land has been a common practice across the globe and the sewage sludge generated from wastewater treatment plants is also used as fertilizer in agricultural soils. Therefore, it is necessary to be aware of possible exposure pathways of these NPs, especially in the perspective of wastewater treatment and their impacts on the agro-environment.
1. Definition
1. Introduction
The tremendous increase in the production and consumption of titanium dioxide (TiO2) nanoparticles (NPs) in numerous industrial products and applications has augmented the need to understand their role in wastewater treatment technologies and their impacts on the agro-environment.
2. Introduction
TiO2 NPs are one of the most extensively used NPs in different sectors [1]. For example, TiO2 NPs are widely used in the agriculture sector for different purposes such as nano-pesticides and nano-fertilizers to introduce sustainable agricultural practices [2]. The availability of these nano-based agrochemicals in the market is expected to rise in near future [3]. Similarly, the use of TiO2 NPs has also gained the utmost importance in other fields, and eventually from different sources, the inevitable release of these NPs into the environment is obvious either through a direct or indirect route. For example, in 2008, the first evidence of TiO2 NPs leaching (3.5 × 107 NPs per L) into the aquatic environment from facade paints was reported [4]. In 2011, TiO2 NPs were first detected in effluents of wastewater treatment plants, which were discharged into freshwater bodies where these NPs can cause unknown ecological risks [5]. TiO2 NPs have also been observed to detach from some textiles and paints due to washing or weathering and to run into wastewater treatment plants [6][7] and especially in sewage sludge reaching the approximate concentration of 2 g·kg−1 [8]. Sewage sludge is commonly employed as soil fertilizer in agriculture at the rate of approximately 3 tons per hectare (on a dry weight basis) annually [9][10][11] and become an ultimate source of TiO2 NPs dissemination in agricultural soils. However, the overall concentration of these NPs in the environment through direct exposure route will be much higher than the indirect release. Interestingly, in both soil and water medium, TiO2 NPs can be used for purification purposes due to their unique characteristics of photocatalysis in the presence of ultraviolet (UV) light [12][13]. below illustrates the brief overview of TiO2 NPs applications, their role in wastewater treatment and their impacts on agro-environment.
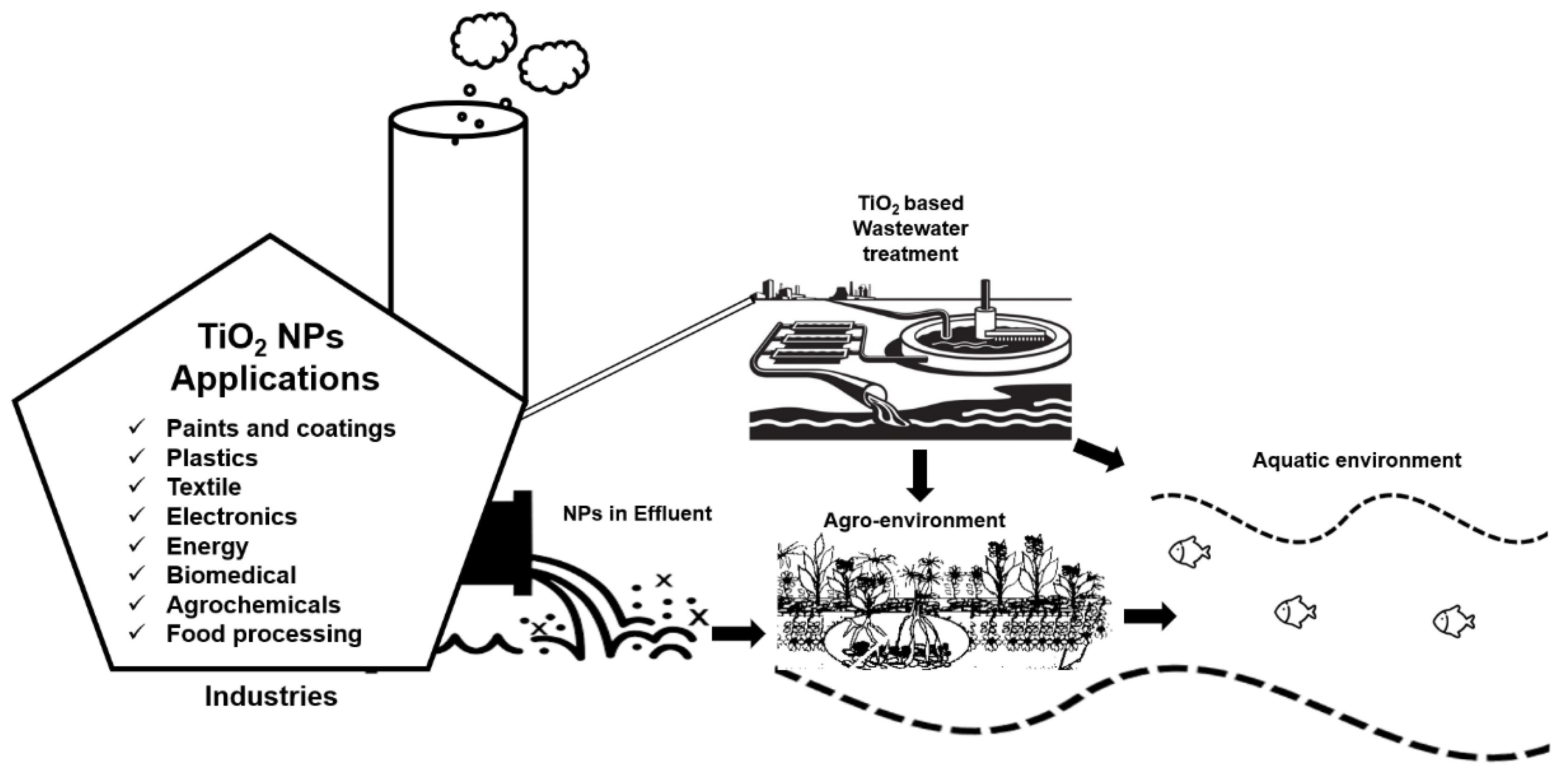
Figure 1. Illustration of the wide range of TiO2 NPs applications from industries, their release into the wastewater, and their possible exposure routes towards the agro-environment.
With the onset of industrialization, there has been a steady increase in the types and amount of pollutants released in the environment. These environmental problems have garnered much attention on the global scale, especially water scarcity. Global water scarcity is a temporal and graphical mismatch between freshwater resources and the world’s water demand. The increasing world population and urban industrialization have made water scarcity more alarming as shown in , predicting the gap between supply (4200 billion m3) and demand (6900 billion m3) of freshwater in 2030. A major proportion of this water is used for the agriculture sector and then for the industrial sector.
Figure 2. Comparison of current and future water demand, Reproduced with permission from
[14], published by McKinsey & Company, New York, NY, USA, 2009.
With a growing world population, an ever-increasing demand for food production and potable water is questionable. The agriculture sector requires a surplus amount of water for irrigation. To avoid water scarcity issues, the reuse of wastewater is tremendously increasing across the planet. Reusing wastewater is a sustainable strategy to manage natural water resources
[15] . However, the use of untreated wastewater for irrigation is a usual practice in developing countries causing serious threats to the ecosystem as well as human health. Specifically, carcinogenic pollutants pose a solemn threat to agricultural land, irrigated with industrial effluent without any treatment
[16].
The whole world stands as a witness to unintended repercussions caused by rapid industrialization. The wastewater generated from industrial sectors has pronounced effects on humans as well as landmass fertility. Some industrial estates have operational wastewater treatment plants but unfortunately, they cannot handle a large proportion of industrial effluent. To meet the international standards of wastewater discharge, suitable technologies are required for wastewater treatment before discharging to streams. It could help to reduce the burden on freshwater resources by reusing treated water in various industrial processes. Due to the widely used application of nanotechnology, challenges, and opportunities of using engineered nanomaterials (ENMs) in wastewater treatment is a matter of endless concern. Based on the wastewater standards, a technique using TiO2 NPs for resilient pollutants in the context of wastewater treatment has become popular in recent years. Up to date, TiO2 NPs have drawn attention over other photocatalysts in every field of life. Over the last few decades, TiO2 NPs with high photocatalytic efficacy has been tested to reduce the pollution load from various industrial units. The conventional wastewater treatment methods mostly come up with high costs as well as lower efficiencies. However, the advantages of the use of TiO2 NPs (non-toxic, inexpensive, stable, and reusable NPs) appeared as a promising strategy to save the environment from pollution.
3. Impacts of TiO2 NPs in the Agro-Environment
In agro-environment, soil is the main and complex matrix in which analyzing the fate of TiO
2 NPs is a challenging task. Furthermore, the impacts of TiO
2 NPs are difficult to measure in the soil due to the high geogenic background of Ti (≈0.6% of the terrestrial crust). Up until now, modeling studies had helped to estimate the approximate amount of TiO
2 NPs that is accumulating in the environment. According to recent forecasts, TiO
2 NPs sludge treated soils (with 45,000 tons) were observed to be the largest sink for NPs release among different environmental compartments
[4]. The crop plants served as an entry route for NPs’ uptake into the food chain. Presently, there are limited data available about these NPs interactions within the soil matrix. As nanotechnology is emerging in the field of agriculture sector in terms of growing global food production, nutritional contents, quality, food safety, and security
[17]. Besides all these aspects, there are several other applications of NPs in agro-environments such as food processing and production, nano-fertilizer, nano-pesticides, etc. but the important concern arises here is the fate of these NPs.
Scientists have investigated the effects of TiO
2 NPs on the soil–plant continuum and have observed diverse impacts based on different characteristics of NPs, plant species, experimental conditions, and exposure period. For example, , shows the TiO
2 NPs effects on plants with respect to different stages, concentration range, and exposure time. In a recent study, experiments were conducted on growth-promoting rhizobacteria (PGPR) inoculation with and without TiO
2 NPs in peat soil under the three stress situations. TiO
2 NPs were reported to enhance the performance of growth-promoting rhizobacteria which further promotes the solubilization of insoluble phosphates
[18]. A grassland soil was treated with TiO
2 NPs at the rate of 0, 500, 1000, and 2000 mg kg
−1 of soil. These NPs were observed to negatively affect the soil bacterial communities after 60 days of exposure
[19]. TiO
2 NPs effects on several bacterial taxa were also studied using incubated soil microcosms having concentrations range of TiO
2 NPs 0, 0.5, 1.0, and 2.0 mg g
−1 soil. Of the identified taxa that exist in all samples, 9 taxa were found to be positively correlated with TiO
2 NPs, 25 taxa were negatively correlated whereas 135 taxa were not affected by TiO
2 NPs
[20]. In another study, TiO
2 NPs effects were investigated at concentrations ranging from 0.05 to 500 mg kg
−1 dry soil on different bacterial communities. The abundance of ammonia-oxidizing archaea was reported to decrease by 40% in response to TiO
2 NPs whereas
Nitrospira was not affected at all. Furthermore, the abundance of ammonia-oxidizing bacteria and
Nitrobacter were also reported to reduce due to TiO
2 NPs treatments
[21].
Figure 3. Effects of TiO
2 NPs on plants with respect to different stages, concentration range, and exposure time. (
a) represents the effects of TiO
2 NPs on germination % of fennel seeds after short term exposure in a petri dish, the lowercase letters show the level of significance such as ‘a’ represent significant increase in germination percentage at Nano 60 treatment compared to control group. Adapted with permission from
[22], published by ELSEVIER, 2013, (
b) shows the effects of these NPs on lettuce plants after long term exposure of 90 days in soil, Adapted with permission from
[24], published by American Chemical Society, 2015.
TiO
2 NPs (0, 5, 20, 40, 60, and 80 mg/kg) were used to study phytotoxicity and stimulatory impacts on fennel after 14 days of exposure. The mean germination percentage was increased by 76% at 60 mg L
−1, while the mean germination time was decreased by 31% at 40 mg L
−1 [22]. Similarly, in another study, plant shoot-root length was increased by 49% and 62%, respectively at 100 mg kg
−1 of NPs treatment in lettuce after 14 days exposure in soil medium
[23]. Another study was performed using TiO
2 NPs treatments (0, 50–250 mg kg
−1) in soil medium for a period of 90 days. The total dry biomass was observed to increase 1.4-fold and phyto-available phosphorus (P) in soil by 2.2-fold, respectively
[24]. enlists the recent studies conducted for the investigation of TiO
2 NPs effects on different plants.
Table 1. TiO2 NPs applications since 2010 on different plants and their impacts.
Studies have shown the positive effects of TiO
2 NPs on the physiology of red bean plants, leaving no negative biochemical impacts in plants
[48]. Low concentrations of TiO
2 NPs were reported with their positive effects on chickpea cells especially when they were exposed to cold stress. However, TiO
2 NPs especially at 5 mg kg
−1 concentration level was reported to reduce cold-induced damages in sensitive and resistant chickpea genotypes. Such domino effects raised key questions regarding the potential mechanisms. It was supposed that the activation of the defensive mechanisms in chickpea seedlings after the absorption of TiO
2 NPs support the plants in cold stress. These results are quite interesting for further practice in cases of environmentally stressed conditions. These new findings could pave the way to increase the use of NPs especially to improve the cold stress tolerance in major crops
[49]. Furthermore, in future studies, TiO
2 NPs application in combination with fertilizers could be an effective option to search out a way for better application of these agrochemicals in a sustainable way. We further need to explore the potential of nanotechnology by upscaling the present studies by investigating the effects of NPs at different stages in the life cycle of plant species and understand their mechanism of environmental exposure.
4. Conclusions and Future Perspectives
Based on the recent developmental facts related to TiO
2 NPs in wastewater treatment technologies and the agro-environment, the use of TiO
2 NPs will further increase for promising applications in the near future. In wastewater treatment technologies, downstream separation of these NPs after photocatalytic degradation is still a matter of concern which can be minimized by using TiO
2 in photocatalytic reactors either in slurry form or immobilized on a solid substrate. Immobilization might result in loss of potential active sites which could be minimized by adding NMs into the polymeric substrate. The polymer can provide firm anchoring to TiO
2 NPs, however, there is still a chance of NPs leaching into the treated water and reaching the agricultural soils via irrigation. Since the agriculture sector is the backbone of the economy in most countries, studies based on crop improvement using TiO
2 NPs could help to overcome the burden of nutrient deficit in soils providing better crop yield. Apart from the potential benefits of TiO
2 NPs there are also some limitations that we could not ignore. At this stage, we could not claim with surety that the use of NPs is fully safe for human health and the environment or if it is harmful. Risks associated with chronic exposure of these NPs, interaction with flora and fauna, and their possible bioaccumulation effects have not been fully considered yet. The other limitations include the lack of information about a safe range of NPs’ concentration, scalability of research and development for prototypes, industrial production, and public concern about health and safety issues. Detailed investigations are necessarily required to resolve these concerns and provide conclusive statements. We need to optimize the useful concentration levels of TiO
2 NPs for various applications and limit their usage for environmental safety.