2. L-Ascorbic Acid as a Pro-Drug
Given the new interests regarding the pharmacokinetic profile of AA, the attention of researchers and clinicians has also focused on the pharmacodynamic aspects of the molecule.
A mechanism for AA anticancer activity has been proposed, according to which the molecule behaves as a pro-drug for the selective transport of hydrogen peroxide (H
2O
2) into the extravascular space near cancer cells
[6][7][6,7].
Therefore, the peroxide (H
2O
2) would be the effective drug, capable of causing toxicity towards cancer cells through a series of selective cytotoxic mechanisms that involve in depletion of ATP leading to cancer cell death
[8][9][12,13]. AA only at specific pharmacological concentrations (0.3–20 mM), versus physiologic (0.1 mM), is able to determine toxicity toward tumor cells, through the formation of H
2O
2, starting from the radical of AA (ascorbyl radical (Asc •))
[5][6][7][5,6,7]. Based on in vitro and in vivo experimental tests, Levine et al.
[5] and Qi Chen et al.
[5][6][5,6] proposed a mechanism for the generation of H
2O
2, starting from ascorbate mono anion (AscH
−).
The presence of AA in equilibrium with its anionic form (AscH
−), can determine the reduction of the endogenous iron contained in the metal proteins, where Fe
3+ is reduced to Fe
2+ with the formation of Asc • (
REACTION 1). Subsequently the Fe
2+ donates an electron to the molecular oxygen, with the formation of active oxygen including superoxide (O
2 • −) (
REACTION 2) with subsequent dismutation in H
2O
2 (
REACTION 3).
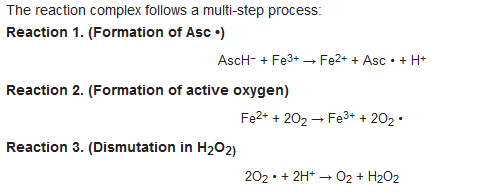
Therefore, the formation of Asc • is closely linked to the presence of metals, hypothetically identified within proteins. The reactivity of AA towards metals has been known for a long time
[10][14], indeed AA is easily oxidized by Fe
3+ ions through an intermediate chelate complex, forming a stable Asc •.
On the other hand, it is also known that the redox-active Fe
2+ ion in the presence of peroxides, initiates a cascade of redox reactions with the formation of ROS (Fenton Reaction)
[10][14]. However, only recent research has hypothesized an in vivo mechanism, in which the electron lost from ascorbate ion (mono anion) selectively induces H
2O
2 formation in extracellular fluids. The peroxide generation process is mainly tissue-specific, as in vivo tests have shown that Asc • and H
2O
2 are mainly detectable in the extracellular fluid but not in the blood
[6][7][6,7]. A series of in vivo experimental tests were conducted to explain the preferential Asc • and H
2O
2 formation in extracellular fluid over blood, after parenteral administration of AA. The results obtained supported the hypothesis that in the blood, the ability to form H
2O
2, starting from Asc •, is very reduced compared to extracellular fluids, because its formation is inhibited by the presence of reducing proteins, not equally distributed in the extracellular space
[4][5][6][7][4,5,6,7].
Red blood cells normally contain large amounts of enzymes capable of preserving hemoglobin from oxidative damage and capable of neutralizing the circulating H
2O
2 in the blood.
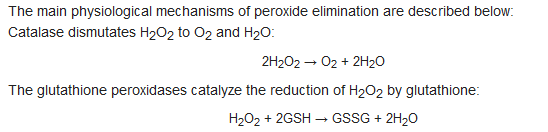
The combined action of these enzymes contributes to an efficient removal of peroxide from the blood and this does not allow to reach systemic doses of H
2O
2 that could be effective against cancer cells.
The data suggest that the half-life of Asc • in blood is also limited and that the process required, to form peroxide, is related to the extravascular compartment.
AA is a hydrophilic molecule subject to oxidation which in oral or parenteral administration, is subject to rapid elimination by the body. The limitations due to the achievement of active Asc • and H
2O
2 concentrations in extracellular tissues, are also related to the pharmacology of the parent molecule AA
[5].
AA is subject to a series of physiological control mechanisms that limit its bioavailability in oral administration, such as intestinal saturation. Alternatively, the parenteral administration, although capable of reaching plasma concentrations higher than those possible with the maximum tolerated oral doses, does not seem able to guarantee a constant efflux into the extracellular fluids. It has been estimated that Asc •, in extracellular fluids, must have a concentration >100 nmol/L to generate H
2O
2 [6][7][6,7] and it is important to note that the presence of Asc • shows a linear relationship with the initial amount of AA present in the blood.
A further observation is related to the exposure of the pharmacological concentrations of the Asc • which must be constant and lasting over time, to develop toxicity in tumor tissues
[6].
In this context it can be assumed that the selective toxicity of peroxides requires high concentrations and sufficiently long times to kill cancer cells.
A further point of interest is linked to the selective toxicity towards cancer cells by H
2O
2.
In conclusion, the key points for a selective toxicity towards cancer cells are the generation of significant quantities of H
2O
2 (mediated by metal-protein catalyst) and by supra physiological concentrations of AA, which must be constant and continuous over time to generate the death of cancer cells.
34. L-Ascorbic Acid in the Body
AA exists mainly in two forms in vivo, the reduced form AscH
2 and DHA (oxidized form), of which the first is by far predominant in the ionic form AscH
− (at physiological pH). Most cell types are able of effectively reducing DHA to AscH
− and thus the total capacity of AA available in the whole organism is considered to be the combined pool of AscH
− and DHA
[11][17]. The turnover of AA is therefore particularly linked to the catabolism of DHA which occurs by hydrolysis to 2,3-Diketogulonic acid and decarboxylation to l-Xylonate and l-Lyxonate, both of which can enter the pentose phosphate pathway for further degradation
[11][17]. The metabolism of AA does not involve conjugation reactions: in contrast to plants, where a number derivatives and analogues, including several glycosides have been identified, only non-conjugated forms of AA are present in mammals
[11][17].
45. Specific Tissue Activation and AA Distribution
Pro-drugs are inactive precursors that are bio-transformed into active metabolites by the action of enzymes or by biochemical mechanisms. Prodrugs can be intentionally designed to overcome pharmacokinetic problems, for example to give the drug site-specific activation in organs or tissues.
To consider AA as a pro-drug, it is important to analyze its site-specific activation in extracellular tissue mediated by metal proteases.
Levine et al.
[5] hypothesized that in the interstitial space of the tumor, AA is led to form its radical (Asc •) starting its mono anionic form (AscH
−). This reaction (
Reaction 1) occurs mainly in extracellular tissues where suitable metal catalysts are present, while in the blood, these reactions are reduced to a minimum.
All the hypotheses discussed above require adequate access for AA to tumors cells with effective distribution throughout the extracellular environment near the tumor.
Therefore, the critical point is related to the distribution of AA from the blood to the tissues.
Below saturation, only cells adjacent to the blood vessel wall accumulate physiological intracellular concentrations and the diffusion zone within the tissues does not extend beyond certain thresholds, even with high plasma AA concentrations
[4].
Cells distant from vessel walls in deep tissues cannot accumulate AA, so supraphysiological levels are required to overcome this diffusion limit.
56. Physiological Role of AA in the Skin
To maintain its elastic, structural, and functional properties, the skin needs numerous substances, including AA. Inside, AA supports important and well-known functions, such as: stimulating collagen synthesis and assisting antioxidant protection against UV-induced photodamages. The skin, therefore, is characterized by physiological concentrations of AA present both in the dermis and in the epidermis
[12][8]. Most of the AA contained in the skin appears to be contained in the intracellular compartments in a concentration of mM order
[12][8].
The physiological distribution of AA in the skin from the plasma occurs via a flow of nutrients from the blood vessels in the dermis to the epidermal layer (
Figure 13).
Figure 13. Enlarged representation of the skin. In pink, the layer of the dermis, supplied by blood vessels that allow the transport of nutrients towards the epidermis in dark purple.
Transport through the skin layers is mediated by specific sodium-dependent vitamin C transporters (SVCTs) which are present throughout the body and are also responsible for transport to other tissues
[4][5][12][11][4,5,8,17].
AA actively accumulates in epidermal and dermal cells via the two sodium-dependent AA transporter (SVCT) isoforms 1 and 2
[12][8].
The presence of this specific transporter suggests that AA has crucial functions within skin cells. Both transporters are hydrophobic membrane proteins that co-transport sodium, driving the uptake of AA into cells and exhibit different absorption kinetics reflecting their different physiological functions.
67. Passive Diffusion of AA and Implications for Its Topical Use
Recent studies suggest that encapsulation in a lipospheric form can promote transport into the lower layers of the epidermis and can ensure greater absorption. However, AA derivatives are not currently used in the field of skin cancers. Both its biodistribution through skin layers and through biological membranes has been little studied and the implications for its topical use are poorly understood. It has long been believed that this hydrophilic molecule only needs protein transporters to cross biological membrane barriers. The distribution of AA within the body requires bi-directional fluxes and, so far, only the AA transporters (SVCTs), facilitating its intake by cells have been identified. However, recent data suggest the implication of a possible passive transport of AA [13][14][18,19]. Experimental tests have shown that AA binds efficiently to the lipid bilayer interface and slowly crosses its hydrophobic core. It has been shown, using lipid membrane models, that AA crosses the lipid bilayer by passive diffusion and that the permeability coefficient depends on its protonation and high concentration gradients [14][19]. The permeability of the deprotonated AA (AscH−) has been determined to be orders of magnitude less than its protonated form (AscH2), so the AA must be protonated at the lipid bilayer interface before entering the hydrophobic region (Figure 24). It has been shown [13][18] that in AA homeostasis, the SVCT transporter is able to generate an AA concentration gradient across the plasma membrane by increasing its intracellular concentration. In turn, the concentration gradient generated by SVCT activity pushes the passive diffusion of AA out of the cell.
Figure 34. Only the protonated form AscH
2 is able to cross biological membranes by passive diffusion. The monoanionic form is unable to pass lipid membranes.
These studies show that the AA must be protonated at the interface of the lipid bilayer, before entering the hydrophobic region. Therefore, to ensure its passive diffusion, the concentration and pH are decisive chemical-physical parameters. In light of all the above considerations, we examine the potential clinical translation of AA by focusing on skin cancers and in particular epitheliomas.
79. Etiology and Treatment of NMSC
Regarding the treatment of NMSC, certainly the complete surgical excision or RT, offers the highest and most reliable rates of disease control and a better prognosis.
In the treatment of BCC, in addition to radiotherapy were described further conservative treatments although with higher rates of persistence/ recurrence, that are photodynamic therapy, cryotherapy, curettage, electrodissection, use of intralesional alpha interferon, and the topical use of imiquimod. In the primary BCC for non-surgical treatments percentages of persistence or recurrence are reported: from 0% to 21% for cryotherapy; from 3% to 42% for curettage and electro-desiccation; from 2% to 30% for intralesional alpha interferon; 0% to 3% for photodynamic therapy; from 7% to 16% for radiotherapy; from 2% to 10% for surgical excision; from 7% to 21% for topical use of 5-fluorouracil; from 0% to 31% for topical use of imiquimod. In the literature, many studies certified the utility of the topical use of imiquimod and 5-fluorouracil fort the treatment of NMSC.
Nevertheless, we found three studies regarding the use of AA for the treatment of NMSCs, including SCCs.
These three studies provide us with an overview for a potential new treatment and give us empirical confirmation of the mechanism of AA in the skin. In this context, AA shows a not yet described mechanism of action for NMSC skin cancers and new research in this area could be considered for the development of new drugs, based on a mechanism of action similar to AA.
AA-based treatment could be used in addition to established therapies or could represent an additional therapeutic option. Topical treatments used for these cancers are mainly encouraged due to the high incidence of NMSC, especially in the elderly population. The high costs associated with the management of this category of patients related to hospitalizations and surgery mean that there is interest in the search for simpler and cheaper treatments.
Furthermore, a simple topical treatment that can be performed before surgery, to favor a more conservative approach, would mean a great improvement in clinical practice. NMSCs mainly affect the head and neck area and can lead to major mutilations (complete nose-ear resection), therefore, reducing the size of the lesions would lead to less disfigurement and improve the patient’s condition.
Although NMSCs can be effectively treated with traditional treatments, there is great interest in new topical treatments related to the reasons discussed above.
810. L-Ascorbic Acid for Topical Use in NMSC
The clinical cases [15][16][17][9,10,11] seem to confirm the rationale presented by Levine et al. [5] and Qi Chen et al. [6][7][6,7], according to which, AA would act as a pro-drug and how its pharmacokinetic plays a central role. These studies take into account the treatment of skin disease, considering the limitations related to biodistribution previously described and providing an empirical approach for topical treatment of BCC and SCC of the skin. It is therefore of interest to analyze how the chemical-physical characteristics of this formulation, can find confirmation in its clinical use. In this context, some considerations can be made. A topical formulation, with adequate concentrations of AA and pH values, can be prepared to obtain the best characteristics to ensure transport into the layers of the skin. AA in topical administration can generate Asc • and H2O2 directly in extracellular tissue, close to tumor cells, without implications related to its pharmacokinetics.
Asc • and H2O2 can be formed by site specific activation, mediated by metal proteins present in the tissues, bypassing the inhibitory action of the blood. Furthermore, continuous exposure over time to peroxide, capable of determining cytotoxicity towards tumor cells, would be more easily achievable than systemic administration.
AA is able to passively distribute itself across membranes [13][14][18,19] and therefore its transcutaneous absorption is directly influenced by the pH of the environment and the pKa of the molecule. It is known that the ionization of a molecule influences its diffusion, because cell membranes have a greater permeability towards its non-ionized form than the less fat-soluble ionized one. Generally, an acid vehicle improves the absorption of molecules with acid characteristics in the undissociated state and the concentration gradients determined by ion trapping can theoretically be very large if the difference in pH between the compartments considered is large. The difference in pH between the compartments plays a role in the absorption of drugs and in the case of acid topical formulations, the difference with the pH of the skin could determine a further contribution for its passage through the stratum corneum.
Activation of AA (as a pro-drug) should only occur in extracellular fluids and the formulation should not contain potentially interfering metal species to promote radical formation. The formulations used in the clinical cases considered were prepared extemporaneously [17][11] without excipients and with highly purified H2O. It can be hypothesized that the presence of potential metal catalysts can initiate the auto-oxidation of AA, favoring the formation of its radical before tissue activation.
AA tends to oxidize easily in light and in the presence of oxygen, so its use is limited by these conditions.
Furthermore, lipophilic derivatives could also be considered, to ensure better absorption, or derivatives less susceptible to oxidation. However, it is not clear whether chemical modifications of the AA, can interfere with the formation of its radical. Further studies on biochemistry and optimization of formulations will be needed in the future to overcome problems related to its stability.
A further step will be to investigate how the skin condition may affect the outcome of the treatment. The skin is in fact a dynamic organ and its characteristics can vary due to various factors such as: damaged skin, keratinization, metabolic states, but also age and diseases.
The dynamism of the skin has also been addressed by recent evidence that has linked the circadian clock to changes in skin physiology such as pH and permeability
[18][19][30,31]. By exploiting the daily variations of circadian cycles in the functions of the epidermal barrier, the transcutaneous absorption of locally applied drugs could be improved.
In light of the above, it remains to be analyzed whether physiological, pathological, and circadian changes can lead to changes in AA absorption, distribution, and mechanism of action.
Studies have suggested that AA could be used as a treatment for BCC and SCC of the skin. The use of a topical formulation of AA, in a context of increasing incidence of these diseases, could be part of current therapeutic strategies. In conclusion, further studies are needed to understand the potential role of this treatment. Although the cases discussed here are preliminary clinical studies, it is important to consider them as a first step in the development of new topical therapies for skin cancers diseases.