3,5,7,3’,4’-Pentahydroxyflavone or quercetin dihydrate (C
15H
10O
7) is the most abundant flavonoid in human dietary nutrition. It is found mostly in flowers, apples, seeds of tomatoes, berries, fennel, tea leaves, nuts, onions, broccoli, pepper, lovage, and shallots
[65][66][65,66]. Quercetin acts as the base for the formation of other flavonoids skeletons, such as naringenin, rutin, and hesperidin
[67]. Quercetin is involved in several biological actions such as: glucose homeostasis; insulin sensitizing and secreting; glucose utilization in peripheral tissues; the inhibition of intestinal glucose absorption
[68]. Quercetin intake is inversely associated with the prevalence of T2DM in the Chinese population which suggests its preventive activity against T2DM
[69][70][69,70]. A recent systematic review and meta-analysis of animal studies showed that quercetin decreases serum levels of glucose at doses of 10, 25, and 50 mg/kg of body weight
[71]. Quercetin extracted from berries induced an insulin independent 5
’ adenosine monophosphate-activated protein kinase (AMPK) pathway which slows the oxygen consumption of adenosine diphosphate by stimulating GLUT 4 translocation and expression in isolated mitochondria. This mechanism has a similar activity as metformin (medication used to treat type 2 diabetes)
[72]. The antidiabetic action of quercetin involves the reduction of lipid peroxidation, glucose absorption by GLUT2, and the inhibition of insulin dependent activation of phosphoinositide 3-kinases (PI3K)
[73][74][73,74]. In addition to this, quercetin and its derivatives (
Table 1) stimulate a glucose uptake in muscle cells, and activate AMPK
[75]. Treating streptozotocin (STZ)-induced diabetic rats with quercetin decreases the activity of glucokinase, hyperglycemia stimulating GLUT 4, hepatic gluconeogenesis, and glycogenolysis while it increases glucose liver uptake
[76]. Quercetin supplementation for two weeks lowered the blood glucose level, upregulated the expression of genes involved in cell survival and proliferation in a liver, and enhanced the serum insulin in STZ- induced diabetic mice
[77]. An injection of quercetin intraperitoneally into STZ- induced diabetic rats, reported a decrease in hyperglycemia, plasma cholesterol and triglycerides, and an improve glucose tolerance and hepatic glucokinase activity
[78]. The co-treatment of quercetin and sitagliptin (a selective dipeptidyl peptidase-IV inhibitor) demonstrated an improvement in its oxidative and inflammatory status, metabolic profile, glycemic control, β-cells function, and islet structure in STZ- induced DM in rats
[79]. Quercetin blocks the activities of a tyrosine kinase inhibitor, which has shown an effect against diabetes (
Figure 3). The regulatory effect of quercetin to nuclear factor kappa-light-chain-enhancer of the activated B cells (NF-κB) also helps in improving glucose stimulated insulin secretion
[80] (
Figure 3).
Figure 3. Flavonoids as anti-diabetic agents: Modes of Action. Aberrant signaling pathways (Glucose transporter, hepatic enzymes, beta cell apoptosis, PPARS, AMPK, Tyrosine kinase inhibitor, and NF-κB) and pathway components targeted by flavonoids (highlighted in green). Flavonoids have a wide range of anti-diabetic actions where one flavonoid could target multiple pathways. These phytochemicals can enhance or suppress (green and red lines respectively) the activity of GLUT 4 translocation, glucose uptake by the tissue, and hepatic enzymes activities; causes a decrease in apoptosis and tyrosine kinase inhibition that improves the pathogenesis of diabetes (see text for detailed modes) of action for flavonoids mentioned). For abbreviation, see abbreviation list.
Table 1. Representative flavonol and their underlying anti-diabetic effects.
Flavonoid Subclass |
Name of Flavonoid |
Structure of Flavonoid |
Dietary Source |
Metabolites Produced from Flavonoids |
Function of Flavonoids |
Mechanism of Action |
Model Used |
References |
In Vivo |
In Vitro |
Flavonol |
1. Rutin |
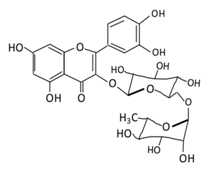 |
Oranges, grapes, limes, lemons, berries and peaches. |
Metabolization depends on intestinal bacteria:
(A) Bacillus 52 and Bacteroides 45 produce: Quercetin-3-O-glucoside and Leucocynaidin.
(B) Bacteroides 42 and veillonella 32 produces: Leucocynaidin.
(C) Bacteroides 22 hydrolysis produce: Quercetin-3-O-glucosie |
(A) Antihyperglycmeic effect:
(B) Hypolipemic effect |
Inhibit α-glucosidase and α-amylase which reduce the absorption of glucose in small intestine
Decrease G6Pase, PEPCK, glycogen phosphorylase, and fructose-1,6-bisphosphatase enzymes in liver and kidney
Decrease the level of caspase 3 and increase the level of Bcl-2 which shows an anti-apoptotic activities
Reduce the level of hemoglobin A1C (HbA1c)
Activate the synthesis and translocation of GLUT4 that stimulate glucose transport to soleus muscle tissue
Increase hexokinase activity in liver
Improve the morphology of islets of Langerhans
Reduce serum LDL, VLDL, triglyceride
Inhibit lipid peroxidation
Increase serum level of HDL
Activate the expression of PPAR-γ which improve glucose uptake and insulin resistance |
Streptozotocin
induced diabetic rats
Type 2 diabetic rat
Streptozotocin
induced diabetic
wistar rats |
Streptozotocin
diabetic tissue |
[81][82][81,83] |
2. Fisetin |
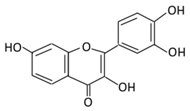 |
Onion, strawberries, and persimmon |
(A) Glucuronide of fisetin (M1)
(B) Glucuronide (M2)
(C) Methoxylated metabolites of fisetin (M3) |
(A) Antihyperglycmeic effect |
Inhibit gluconeogenesis by inhibiting pyruvate transport into mitochondria
Decrease glycogen breakdown which prevent hyperglycemia
Reduce blood glucose, Hb1Ac, IL-1β, and NF-κB p65 unit
Reduce the activity of glucose glucose-6-phosphate dehydrogenase activity |
Streptozotocin induced diabetic
rats |
|
[83][84][107,108] |
3. Kaempferol |
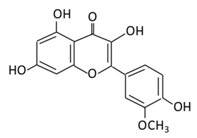 |
Cruciferous vegetables, tea, grapefruit, edible berries, and Gingko biloba L. |
(A) Kaempferol-3-O-glucoside
(B) Kaempferol-3-O-diglucoside |
(A) Antihyperglycmeic effect:
(B) Hypolipemic effect |
Reduce serum glucose level and fasting blood glucose level
Decrease the level of caspase 3 activity in β-cells
Inhibit cellular apoptosis by improving anti-apoptotic Akt activities
Improve cAMP signaling and insulin synthesis and secretion
Improve glucose uptake by soleus muscles
Reduce lipid peroxidation
Decrease PPARγ expression through AMPK activity |
Rats
Streptozotocin (STZ)-induced diabetic rats
High fat diet mice |
Pancreatic β-cells |
[85][86][90,91] |
4. Quercetin |
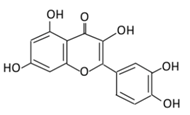 |
Black currants, cherries, apples and chokeberries |
(A) Quercetin-3-O-glucoside
(B) Quercetin -3-O-glucoside-7-O-glucoside
(C) Quercetin-3-O-galactoside
(D) Aglycone |
(A) Antihyperglycmeic effect:
(B) Hypolipemic effect |
Inhibit insulin dependent activation of PI3K
Inhibit GLUT2 which reduces the absorption of glucose in small intestine
Block the activity of tyrosine kinase
Improve GLUT4 translocation through the activation of AMPK
Improve the recovery of cell proliferation
Improve glucose absorption
Reduce lipid peroxidation |
Rats
Streptozotocin (STZ)-induced diabetic rats
High fat diet mice |
Skeletal muscle cells
Hepatocyte
RINm5F β-cells |
[68][69][68,69] |
5. Isorhamnetin |
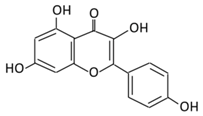 |
Oenanthe javanica, Hippophae rhamnoides, and Ginkgo biloba L. |
(A) Isorhamnetin
(B) isorhamnetin-3-O-galacto |
(A) Antihyperglycmeic effect:
(B) Hypolipemic effect: |
Improve insulin secretion
Increase glucose transporter 2 (GLUT2)
Inhibit adipogenesis |
HFD- induced C57BL/6 mice |
3 T3-L1 cells |
[87][88][102,103] |
6. Morin |
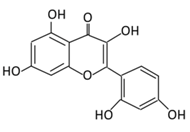 |
Psidium guajava, Prunus dulcis (Almond), chlorophora tinctoria, and fruits |
(A) Morin glucuronides
(B) Morin sulfates |
(A) Antihyperglycmeic effect:
(B) Hypolipemic effect. |
Reduce hepatic NF-κB activation
Reduce G6Pase and FDPase enzymatic activities
Increase hexokinase and G6PD enzymatic activities
Improve hyperglycemia, insulin resistance, and glucose intolerance
Reduce lipid peroxidation
Reduce hyperlipidemia
Normalize the profile of lipid and lipoprotein |
Streptozotocin (STZ)-induced diabetic rats
High fructose fed rats
HFD-STZ induced type 2 diabetic rats |
Rats hepatocyte |
[89][90][114,115] |
2.1.2. Rutin
Rutin is extracted from plants, such as oranges, lemons, grapes, peaches, limes, and buckwheat
[81]. Rutin is also known as glycosylated quercetin, sophorin, and quercetin-3-
O-rutinosie
[91][82]. The anti-diabetic effects of rutin includes the reduction of carbohydrates absorption from the small intestine, the improvement of glucose uptake by tissues, the suppression of tissue gluconeogenesis, the activation of insulin secretion from β-cells, and the protection of the islets of Langerhans from degenerative changes. Rutin also lowers the formation of reactive oxygen species, advanced glycation end-product precursors, sorbitol, and pro-inflammatory cytokines
[82][83]. Several experimental studies evaluated the hypolipemic and antihyperglycemic effects of rutin
[92][84] (
Table 1). The oral or intraperitoneal administration of rutin (50 mg/kg or 100 mg/kg) into a STZ model of type 1 diabetic rats significantly decreased glycated hemoglobin (HbA1c) and fasting blood glucose (FBG)
[93][85]. When diabetic rats were fed with 100 mg/kg rutin, a significant increase in insulin levels and carbohydrate metabolic enzymes activity occurred. Further, the results showed a significant reduction in the level of plasma glucose
[94][86]. The administration of rutin activates hepatic enzymes involved in the gluconeogenic and lipid metabolic process, such as hexokinase (
Figure 3)
[95][87]. The flavonoid also decreases significantly the level of urine protein, blood urea nitrogen, oxidative stress intensity, and fasting blood glucose. The treatment of rutin showed anti-apoptotic activities by increasing the activity level of B-cell lymphoma 2 (Bcl-2) and decreasing the level of caspase-3 in diabetic retina (
Figure 3)
[96][88]. Compared with other flavonoids like boswellic, quercetin, and ellagic acid, rutin was the most active flavonoid in reducing FBG, serum lipids, and improving glucose tolerance
[97][89].
2.1.3. Kaempferol
3,4,5,7-Tetrahydroxyflavone is a nontoxic flavonoid that is abundant in grapes, apples, onions, tomatoes, beans, kale, broccoli, potato, tea, and spinach
[85][90]. Kaempferol has neuroprotective, antimicrobial, antioxidant, anti-inflammatory, and anticancer effects
[86][91]. The extracts of kaempferol from
Bauhinia forficate leaves reduce hyperglycemia and enhance glucose uptake, mimicking the action of insulin
[98][92]. In vitro studies confirmed that treating with 10 μM of kaempferol enhances cellular viability and represses apoptosis
[99][93]. Kaempferol has several antidiabetic effects, like improving AMP activated cellular protein expression and activation, reducing cellular apoptosis by suppressing caspase 3 activities, and increasing the production and secretion of insulin from β-cells
[100][94] (
Figure 3). In addition to this, kaempferol enhances glucose uptake by the cells through protein kinase C and PI3K pathways, and the synthesis of new glucose transporters
[101][95]. The oral administration of kaempferol significantly decreased serum HbA1c levels, fasting blood glucose, and increased insulin resistance. This flavonoid decreased the genetic expression of PPARγ mediated through regulating AMPK activation
[102][96] (
Table 1). Moreover, kaempferol improved DM in STZ-induced mice through the promotion of glucose metabolism in skeletal muscle, and the suppression of hepatic gluconeogenesis
[103][97]. In another study, kaempferol attenuated diabetic nephropathy in NRK-52E and RPTEC cells via suppressing RhoA/Rho-kinase mediated pro-inflammatory signaling (i.e., TNF-α, IL-1β, and TGF-β1)
[104][98]. Like kaempferol, resveratrol is a natural phenol found in grapes, blueberries, and peanuts with potent antioxidant and anti-inflammatory activities that could effectively prevent diabetes
[105][106][99,100].
2.1.4. Isorhamnetin
An
O-methylated bioactive compound is found commonly in medical plants, like
Oenanthe javanica (Chinese celery, Japanese parsley, blume, minari in Korean),
Hippophae rhamnoides (known also as sea- buckthorn), and
Ginkgo biloba (commonly known as ginkgo)
[107][101]. This flavonoid has anti-obesity and anti-diabetic effects
[56]. The oral administration of isorhamnetin for 10 days into a streptozotocin
-induced model of diabetes (STZ) at a dose of 10 mg/kg or 20 mg/kg showed an effective reduction in oxidative stress and hyperglycemia. The anti-diabetic effect of isorhamnetin is, not only limited to reducing the blood glucose level, but also it helps in reducing the accumulation of sorbitol level on rat lenses, the sciatic nerve, and red blood cells.
[87][102]. An experimental study proposed that isorhamnetin glycoside has several effects on diabetes, like stimulating insulin secretion, the expression of enzymes involved in lipid metabolism, and the expression of endoplasmic reticulum stress markers
[88][108][103,104].
2.1.5. Fisetin
3,7,3’,4’-Tetrahydroxyflavoneis found abundantly in fruits and vegetables like apples, grapes, persimmon, cucumber, onions, and strawberries
[109][110][105,106]. Fisetin possess anti-diabetic, anti-inflammatory, and neurotrophic effects
[83][107]. The oral treatment of fisetin in a dose of 10 mg/kg for 30 days decreased Hb1Ac, blood glucose levels, and the expression of the gluconeogenic genes protein level, while it increased the concentration of plasma insulin
[84][108]. In an in vivo study, the results showed that treatment with fisetin significantly reduced the level of NF-κB p65, Hemoglobin A1C (HbA1c), serum nitic oxide (NO), and blood glucose
[111][109]. Fisetin also inhibits high glucose induced cytokine production in monocytes which could prevent diabetes
[112][110]. The anti-diabetic effects of fisetin on hepatic enzymes include enhancing the activities of hexokinase, while reducing the activities of glucose 6 phosphate dehydrogenase (G6PD) and glucose 6-phosphatase (G6Pase) (
Figure 3). Moreover, fisetin improves glucose homeostasis by attenuating carbohydrate metabolism enzymes in STZ diabetic rats
[113][111]. Fisetin has been reported to improve the development of diabetic cardiomyopathy in STZ- induced DM rats by improving hyperglycemia/hyperlipidemia-mediated oxidative stress, the inflammation processes, and the programmed cell death
[114][112]. Preclinical evidence illustrated the therapeutic potential of fisetin in diabetic neuropathy through the modulation of NF-κB and Nrf2 signaling pathways
[115][113].
2.1.6. Morin
A natural flavonoid, morin, is found mostly in traditional medical herbs, like
Prunus dulcis, Chlorophora tinctoria L., and fruits such as guava and figs
[89][114]. The oral administration of morin for 30 days in animal models resulted a significant improvement in glucose tolerance, hyperglycemia, and insulin resistance
[90][115]. Diabetic rats were reported to have declined lipid peroxides and antioxidant levels after the treatment with morin
[116]. Morin effectively decreased the level of inflammatory cytokines, like IL-6 and TNF-α, which proves its anti-inflammatory effects
[117]. In animal models, morin recovered leptin sensitivity and hepatic insulin led to the reduction of liver lipid accumulation and hyperlipidemia
[118]. Morin has different effects on hepatic enzymes where it is significantly reduces the activity of G6Pase and Fructose-1,6-diphosphatase (FDPase), while enhancing the activity of hexokinase and G6PD
[119] (
Figure 3).
2.2. Flavanones
Flavanones are known as di-hydroflavones and they are characterized by an oxidized, saturated carbon ring. Flavanones are widespread in citrus fruits and known for their free radical scavenging ability and antioxidant activity
[64].
2.2.1. Hesperidin
5,7,3’-Trihydroxy-4’-methoxyflavanone, a saturated oxidized aglycon, is found abundantly in citrus fruits, such as limes and lemons, tomatoes and cherries
[120]. The effects of hesperidin and its glycoside (
Table 2) are not limited to diabetes, but also have vascular, neuroprotective, anti-allergic, anti-inflammatory, anticarcinogenic, and antioxidant effects
[121]. A study in db/db C56BL6 mice showed that hesperidin supplementation to the regular diet helps in regulating the activity of gluconeogenesis and glycolytic hepatic enzymes, and in improving hyperglycemia
[122]. In db/db mice, the flavonoid has a very effective machinery, like increasing triglyceride fecal excretion and inhibiting lipid metabolizing enzymes which enhances the lipid metabolism activities
[123]. Hesperidin effectively lowers blood glucose levels by upregulating GLUT 4 translocation and PPARγ
[124]. Hesperidin supplementation showed a decrease in glucose 6 phosphatase (G6Pase) activities in STZ- induced diabetic rats, which diminish glucose exports from the cells by a glucose transporter membrane protein
[125] (
Figure 3). A dose of 10 g/kg diet of hesperidin treatment decreases glucose levels by altering glucose regulating enzyme activities
[126]. The administration of hesperidin and hesperetin together have different effects on lipid and glucose metabolism and show lipid lowering activities
[56]. Hesperidin also positively regulates the α-Klotho/FGF-23 pathway in STZ- induced DM rats, which demonstrate positive effects on diabetic toxicity in the liver and kidney
[127].
64].
2.3.1. Apigenin
5,7,4’-Trihydroxyflavone is a phytoestrogen aglycoge found mostly in nuts, vine spinach, oranges, celery, garlic, tea, oregano, carrot, and chamomile
[147]. In alloxan induced insulin dependent diabetic mice, the oral administration of apigenin for 10 days reduced hepatic antioxidants, like catalase, glutathione, and superoxide dismutase. The treatment with apigenin helps in reducing hyperglycemia, serum cholesterol and G6Pase activities in the liver (
Figure 3)
[148]. In STZ- induced diabetic rats, the administration of apigenin (4 mg/kg) showed a significant anti-hyperglycemic effect
[149]. Apigenin treatment could prevent induced apoptosis through the inhibition of NF-κB activation
[150]. In HepG2 hepatocytes, apigenin enhances the phosphorylation of AMPK. This effect of apigenin is 200 times more potent than metformin
[49]. The enhancement of GLUT4 translocation upon the treatment with apigenin suggested its effect on lowering blood glucose
[151]. Apigenin administration improved oxidative damage of pancreatic β-cells in STZ-induced rats by lowering cellular DNA damage, ROS production, protein carboxylation, lipid peroxidation, and restored cell apoptosis
[152]. The administration of apigenin improved STZ-induced diabetic nephropathy through MAPK-NF-κB-TNF-α and TGF-β1-MAPK-fibronectin signaling
[153].
2.3.2. Luteolin
5,7,3’,4’-Tetrahydroxyflavone is a well-known anti-inflammatory and antidiabetic agent
[154]. It is abundantly found in fruits and vegetables like cabbage, onion leaves, celery, carrots, parsley, peppers, broccoli, and apple skin
[155]. Luteolin was reported to initiate insulin action and to enhance the expression of PPARγ target genes (
Figure 3) in primary mouse adipose cells
[156]. In damaged pancreatic cells, β cells, this flavonoid improves insulin secretion in uric acid by decreasing micro-autologous fat transplantation (Maft), a trans activator of insulin gene through NF-κB signaling pathway
[157]. The anti-diabetic effects of luteolin (LU) and luteolin-7-
O-glucoside (LUG) improve blood glucose, HbA1c, insulin, and HOMA-IR levels, and inhibit lipid synthesis
[158]. Luteolin improves insulin resistance and adipose tissue inflammation by altering M1-like macrophage polarization in adipose tissue
[159].
2.3.3. Tangeretin
5,6,7,8 4’-Pentamethoxyflavone is a flavonoid prevalent in citrus fruits, such as oranges, citrus peel of tangerine, and mandarins
[160]. The administration of tangeretin (200 mg/kg) in HFD-induced obese mice reduced blood glucose, total cholesterol, body weight and regulated adipocytokines, like leptin, IL-6, and adiponectin
[161]. Treating diabetic rats with tangeretin (100 mg/kg) for 30 days reduced glucose plasma levels, Hb1Ac, and enhanced glycolytic enzymes, the level of insulin and hemoglobin significantly
[162]. In 3T3-L1 preadipocyte, tangeretin increases the secretion of the insulin sensitizing factor while decreasing the secretion of the insulin resistance factor
[163]. In addition, tangeretin down-regulates STZ-induced programmed cell death in INS-1 cells through the regulation of NF-κB signaling
[164].
2.3.4. Chrysin
5,7-Dihydroxyflavone is found abundantly in honey, fruits, bee pollen, propolis, and medical plants, such as
Passiflora caerulea L. and
Tilia tomentosa [165]. This flavonoid is an analog to apigenin but with lower bioavailability due to rapid excretion and metabolism
[166]. Chrysin treatment in STZ-induced rats reported an elevation of glucose, MDA, TG, TC, LDL-C and a reduction of HDL-C, total protein, SOD, CAT, and GST
[167]. The treatment with chrysin demonstrated an improvement in renal pathology and suppressed collagen-IV protein expressions in renal tissue
[168]. In HFD/STZ-induced diabetic rats, chrysin significantly prevented the development of diabetic neuropathy (DN) due to the reduced level of pro-inflammatory cytokines in the serum
[169]. Chrysin treatment decreases lipid peroxidation, glucose levels and increases insulin levels in diabetic rats
[170]. The data suggest that chrysin has anti-diabetic and antihypertensive effects
[171].
Table 3. Representative flavones and their underlying anti-diabetic effects.
Flavonoid Subclass | Name of Flavonoid | Structure of Flavonoid | Dietary Source | Metabolites Produced from Flavonoids | Function of Flavonoids | Mechanism of Action | Model Used | References |
---|
In Vivo | In Vitro |
---|
Flavones | 10. Baicalein | 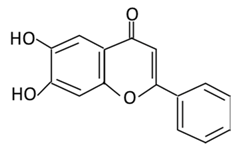 | Scutellaria lateriflora L, and Scutellaria baicalensis Georgi | In Intestine: Baicalin will be converted into Baicalein and then absorbed rapidly.
In the circulation: Baicalein will be converted to Baicalin | (A) Antihyperglycmeic effect:
(B) Hypolipemic effect | Reduce the level of level of hemoglobin A1C (HbA1c)
Suppress the activation of NF-κB
Improve glucose tolerance and insulin secretion from pancreatic cells
Improve viability of clonal β-cells which improves the production of NADH and NADPH
Protect against β cells apoptosis
Increase hexokinase activity in liver
Activate MAPKs signaling pathway which reduce the effect of insulin resistance by phosphorylating Akt and IRS-1 and dephosphorylate NF-κB
Suppress fatty acid synthesis | Obese diabetic mice
Type 2 diabetic rats | CA1 hippocampal neurons | [172][173] |
11. Luteolin | 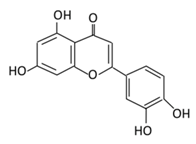 | Parsley, broccoli, onoins leaves, celery, cabbages, apple skins, carrots, and peppers | Metabolization is medicated by UGTs and COMTs to produce:
(A) Luteolin-7-glucuronide (Glucuronidated)
(B) Luteolin-4-glucuronide
(C) Chrysoeriol/diosmetic (Methylated)
(D) Luteolin monoglucuronide (Major form in human serum |
Inhibit glucose uptake by inhibiting sodium glucose co-transporter
Activate AMPK pathway which increase insulin sensitivity and glucose tolerance
Reduce membrane lipid peroxidation
Prevent apolipoprotein B overproduction and dyslipidemia
Induce hypolipidemic activity |
Streptozotocin (STZ)-induced diabetic rats High fat diet fed mice
LDL receptor null mice
Male Sprague-Dawley rats |
INS-1E cells |
[128][129][133,135] |
9.Eriodictyol |
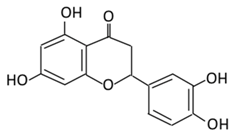 |
Lemon, Torr, Eridictyon californicum, Millettia duchesnei De Wild, and Eupatorium arnottianum |
(A) Monoglucuronide M1 in the liver microsome
(B) Monoglucuronide M2 in the liver microsome |
(A) Antihyperglycmeic effect:
(B) Hypolipemic effect: |
Suppress oxidative stress
Decrease Intercellular Adhesion Molecule 1 (ICAM-1), Vascular endothelial growth factor (VEGF), retinal TNFα, and Endothelial NOS (eNOS).
Reactivate Akt phosphorylation
Reduce lipid peroxidation
Up-regulate the expression of PPARγ2
Up-regulate adipocyte- specific fatty acid binding protein |
Streptozotocin
induced diabetic
rats (0.2%) |
HepG2 cells
Differentiated 3T3-L1 cells |
[130][131][144,146] |
2.2.2. Naringenin
5,7,4’-Trihydroxyflavanone, a saturated oxidized aglycone, is present abundantly in citrus fruits, such as oranges, tomatoes, and grapefruits
[132][128]. It has a wide range of biological activities, such as antioxidant, antidiabetic, anti-inflammatory, anticancer, and anti-mutagenic abilities
[133][129]. Both naringenin and its glycoside (
Table 2) possess anti-diabetic and anti-obesity activities
[134][130]. An in vitro study presented that naringenin mimics the effect of insulin by decreasing pro-liprotein B secretion in liver cells
[135][131]. The administration of this flavonoid (25 mg/kg) into diabetic rats showed a significant inhibition of α-glucosidase activity which delayed carbohydrate absorption, therefore, reducing postprandial blood glucose levels
[136][132]. In type 2 diabetic mice, naringin could upregulate GLUT4, and regulates the expression of hepatic enzymes involved in gluconeogenesis and glycolysis which improves hyperglycemia
[128][137][133,134]. In an everted rat intestinal sleeve, naringenin was found to inhibit the uptake of glucose by inhibiting sodium-glucose co-transporters in the intestine
[129][135]. The administration of naringenin presented various effects in different diabetic rat models: (i) In STZ- induced diabetic rats, the flavonoid decreased the level of plasma glucose; (ii) in fructose-fed insulin resistance rats, naringenin improved insulin sensitivity; in HFD mice, it helped in the reduction of insulin resistance
[138][139][140][136,137,138]. Treating diabetic mice with 25 mg/kg for 45 days significantly reduced hyperinsulinemia, hyperglycemia, decreased lipid membrane peroxidation, improved hepatic markers, restored the changes in lipid profile, and enhanced the antioxidants activities
[141][139]. Naringenin anti-diabetic effects in diabetic rats were characterized by anti-oxidant, and anti-apoptotic activities which showed the potential of naringenin to limit neurodegeneration and prevent retinal damage in diabetic retinopathy
[142][140].
2.2.3. Eriodictyol
Eriodictyol, present in lemon fruits, significantly controls obesity and diabetes
[143][141]. Recently, eriodictyol was identified as a novel insulin secretagogue in vitro and in vivo which exerts an exclusive glucose-dependent insulinotropic activity via a cAMP/PKA pathway
[144][142]. Moreover, in diabetic rats, eriodictyol supplementation can effectively suppress oxidative stress
[145][143]. The treatment with eriodictyol upregulated the expression of PPARγ2 and the adipocyte-specific fatty acid binding protein
[130][144]. Furthermore, eriodictyol treatment significantly suppressed diabetes related lipid peroxidation
[146][145] (
Figure 3). Recently, eriodictyol was described as a protector of the rat retinal ganglial cells (RGC)-5 from high glucose-induced oxidative stress, inflammation, and cell apoptosis via the activation of Nrf2/HO-1 signaling
[131][146].
2.3. Flavones
The structure of flavones is comprised of an unsaturated carbon ring at C2–3 and a ketone group at C4, but they lack the hydroxylation at carbon 3 if compared to flavonols. They are widely synthesized in flowers, leaves, and fruits
[
|
(A) Antihyperglycmeic effect: |
| (B) Hypolipemic effect | Reduce cAMP response element binding protein and histone acetyl transferase activity of CBP/p300 (NF-κB coactivator) |
| Reduce apoptosis
Up-regulate the espression of synaptic protein which target brain cells
Improve insulin secretion by supressing Maf A through NF-κB signiling pathway
Activate PPAR-γ which targets adiponectin, leptin and GLUT4 genes | Obese mice
Streptozotocin induced diabetic
rats
Diabetic rats | Endothelium cells
Human monocytes cells | [155][157] |
12. Diosmin | 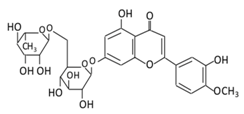 | Citrus fruites, and Scrophularia nodosa L. | (A) Diosmin (Not excreted in urine)
(B) Diosmetin (Not excreted in urine)
(C) Minor metabolites in the form of glucuronic acid conjugate (Excreted in urine) | (A) Antihyperglycmeic effect:
(B) Hypolipemic effect: | Reduce the level of hemoglobin A1C (HbA1c) due to increase in glutathione peroxidase (GPx)
Decrease G6Pase, PEPCK, and fructose-1,6-bisphosphatase enzymes
Reduce plasma glucose and increase plasma insulin by activating anti-oxidant enzymes
Reduce hyperglycemia by inducing β-endorphin
Increase hexokinase and glucose-6-phosphate dehydrogenase activity
Reduce lipid peroxidation | Streptozotocin nicotinamide induced diabetic
rats | | [174][175] |
192][205].
2.5. Anthocyanins
A water soluble, unoxidized, unsaturated flavonoid, anthocyanin, is present abundantly in flowers and fruits. The dietary consumption of this flavonoid is higher compared to other flavonoids. Several studies, both in animal models and cell lines, suggested that anthocyanins have anti-diabetic activities
[56].
2.5.1. Cyanidin
A flavonoid commonly distributed in vegetables, fruits, crops, and other plant-based diets
[193][206], cyanidin, exerts an anti-diabetic effect by inhibiting intestinal α-glucosidase and pancreatic α-amylase
[194][207]. In STZ-induced diabetic rats, cyanidin reversed degenerative changes in β-cells by preventing pancreatic apoptosis and activating insulin receptor phosphorylation
[195][208]. Cyanidin-3-glucoside (C3G), a prominent anthocyanidins in the diet, improved antioxidant status and protected hepatocyte from oxidative stress against high glucose induced damage (HG) (
Table 4)
[196][209].
Table 4. Representative isoflavones, anthocyanins and their underlying anti-diabetic effects.
Flavonoid Subclass |
Name of Flavonoid |
Structure of Flavonoid |
Dietary Source |
Metabolites Produced from Flavonoids |
Function of Flavonoids |
Mechanism of Action |
Model used |
References |
In Vivo |
In Vitro |
Isoflavones |
17. Genistein |
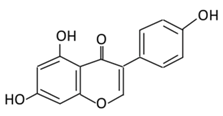 |
Soybeans, kudzu, and fava bean |
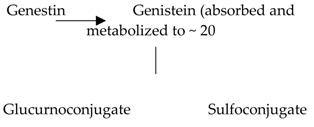 |
(A) Antihyperglycmeic effect:
(B) Hypolipemic effect |
Reduce hyperglycemia through the activity of cAMP/ PKA pathway
Decrease Intercellular Adhesion Molecule 1 (ICAM-1) and p-ERK
Inhibit the activity of tyrosine kinase
Improve glucose intolerance and β-cells mass
Decrease urinary excretion of TBARs |
Streptozotocin (STZ)-induced diabetic rats
Obese diabetic mice
Nongenetictype 2 diabetic mice |
INS-1 cells
Human islet β-cells |
[182][186][195,199] |
18. Daidzein |
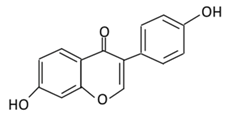 |
Soybeans, nuts, and soy milk |
(A) Daidzin |
(A) Antihyperglycmeic effect: |
Decrease blood glucose, total cholesterol, and AMPK phosphorylation |
Golden Syrian hamsters |
|
[189][202[191],204] |
Anthocyanins |
19. Cyanidin |
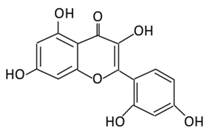 |
Bilberry, blueberry, grapes, blackberries, hawthorn, acai berry, and raspberry |
(A) Anthocyanidin glucuronide conjugates (Major form in urine)
(B) Simple Aglycones (Second major in urine)
(C) Anthocyanidin methyl glucuronide conjugates (8 forms)
(D) Cyanidin-3-glucoside
E) Cyanidin-3-galactoside |
(A) Antihyperglycmeic effect:
(B) Hypolipemic effect: |
Inhibit α-glucosidase and α-amylase which reduce the absorption of glucose in small intestine
Reduce fasting glucose level
Prevent pancreatic apoptosis
Improve antioxidant status which protects hepatocytes from HG-induced damage
Attenuate aortic lipid peroxidation |
Streptozotocin (STZ)-induced diabetic rats
db/db rats
high fat diet fed mice |
Mouse hepatocyte |
[194][196][207,209] |
13. Apigenin | 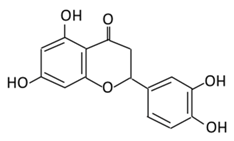 | Onion, oranges, tea, parsley, chamomile, Hypericum perforatum L, wheat sprouts | Metabolization occurs through two phases:
Phase (1): Apigenin produce three monohydroxylated:
a) Luteolin b) Scutellarien c) iso-scutellarein
Phase(2): Luteolin produce:
a) Four monoglucuroconjugates
b) Two Sulfoconjugate
c) One methyl conjugate | (A) Antihyperglycmeic effect:
(B) Hypolipemic effect: |
20.Delphinidin |
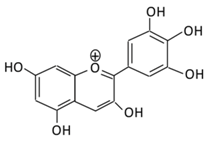 |
Dark grapes, eggplant, berries, red cabbage, carrot, and tomato |
(A) 4′-O- methyl delphinidin 3-O-beta-d- glucopyranoside | Reduce cellular antioxidants |
Attenuate cell damage in pancreatic β-cells
Improve the morphology of the cells
Improve GLUT4 translocation which lowers glucose level
Increase serum cholesterol
Increase lipid peroxidation | Streptozotocin
induced diabetic
rats (0.2%) | HepG2 cells
Differentiated3T3-L1 cells |
(A) Antihyperglycmeic effect: |
Reduce the glycation rate of HbA1c
Prevents diabetes associated injuries such as endothelial cell function | [ |
Diabetic mouse147 |
] |
[197][149] |
[ | 198 | ] | [ | 213 | , | 215 | ] |
|
21.Pelargonidin | 14.Tangeretin | 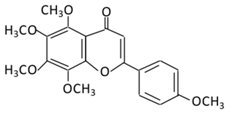 | Poncirus trifoliate L, citrus fruit rinds, and mandarin orange | Metabolization is medicated by CYP1A1 and CYP1A2 to produce:
(A) 4′ hydroxy - 5, 6, 7, 8 tetramethoxyflavone (4′-OH-TMF) | (A) Antihyperglycmeic effect:
(B) Hypolipemic effect: | Reduce blood glucose and HbA1c level
Reduce the secretion of insulin resistance factor
Increase the secretion level of insulin and insulin sensitizing factor
Enhances glycolytic enzyme in the liver
Reduce total cholesterol and adipocytokines level | Rats
Streptozotocin
(STZ)-induced
diabetic rats
High fat diet mice | Pancreatic β-cells | [160][162] |
15. Wogonin | 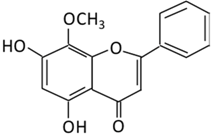 | Scutellaria baicalensis Georgi | (A) Wogonin-7-beta-D-glucuronide (Major metabolites)
(B) Wogonin-5-beta-D-glucuronide | (A) Antihyperglycmeic effect:
(B) Hypolipemic effect: | Reduce hyperglycemia and lipid droplets accumulation in the liver
Increase vascular permeability and the expression of cell adhesion molecules
Activate NF-κB and AMPK pathways
Activate PPARα which has a beneficial effect on lipid metabolism | db/db mice | 3T3-L1 cells | [176][177] |
16. Chrysin | 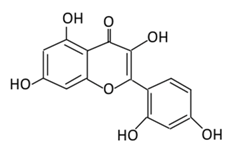 | passiflora caerulea (L,), honey, Tilia tomentosa Moench, and Pelargonium crispum (Berg.) | (A) Chrysin glucuronides (M1)
(B) Chrysin sulfates (M2) | (A) Antihyperglycmeic effect:
(B) Hypolipemic effect: | Reduce the level of pro-inflammatory cytokines that helps in the prevention of diabetic neuropathy
Reduce blood glucose
Improve renal pathology with the suppression of TGF-β, collagen-IV, and fibronectin
Improve insulin level
Reduce lipid peroxidation | | INS-1E cells | [167][169] |
2.4. Isoflavones
Isoflavones are found mostly in legumes, soybeans, and some microbes
[64]. Genistein and daidzein are the major source of isoflavones. They have shown to have an anti-diabetic effect by stimulating insulin secretion from the pancreatic beta cells
[56].
2.4.1. Genistein
5,7,4’-Trihysroxyisoflavone, a naturally occurring soy isoflavone, is present numerously in soy, soybean products, and Chinese plants
[178][191]. Genistein exerts the anti-diabetic effects by enhancing plasma lipids
[179][192]. Genistein supplementation in type 1 diabetes animals led to the improvement of insulin levels and glucose metabolism
[180][193]. An in vivo study found that genistein improved hyperglycemia through promoting cAMP/PKA signaling pathways
[181][194]. The administration of genistein to rats fed with a fructose rich diet showed a protective role on renal malfunction through the modulation of insulin resistance
[182][195]. The supplementation of genistein (0.02% in diet) in non-obese diabetic (NOD) rats showed the onset of diabetes was prevented and glucose homeostasis was improved through the preservation of β cell functions
[183][196]. The beneficial effects were observed in non-generic mouse models ingested with 250 mg/kg of genistein like reduction in the fasting glucose level and β cell mass
[184][197]. In STZ-induced mice, genistein improved glucose tolerance, hyperglycemia, and the level of circulating insulin
[185][198]. Genistein demonstrated an inhibitory effect on tyrosine kinase which dysregulates glucose homeostasis (
Figure 3)
[186][199]. The administration of genistein to mice reduced body weight and improved glucose and lipid metabolism
[187][200]. A transcriptome analysis revealed that genistein could affect the regulation of the hypothalamic circadian rhythms which could provide a novel target for the therapy of diabetes and obesity. Moreover, genistein has a protective effect against inflammation, neuropathic pain, and oxidative stress
[188][201].
2.4.2. Daidzein
7,4’-Dihydroxyisoflavone is a phytoestrogen mainly isolated from nuts, fruits, and soybeans
[189][202]. Daidzein exerts an anti-diabetic effect by enhancing lipid and glucose metabolism
[190][203]. Daidzein has promising therapeutic potential on impaired glucose, lipid metabolism, and vascular inflammation associated with T2DM
[191][204]. Moreover, daidzein treatment in gastrocnemius muscle is effective in decreasing blood glucose, total cholesterol, and AMPK phosphorylation (
Figure 3)
[188][201]. Pure synthetic daidzein administered to hamsters significantly lowered plasma total cholesterol levels and blood glucose compared to the control group
[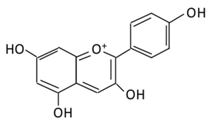 |
Bilberry and |
ficus bengalensis |
Linn |
(A) Pelargonidin- | O | -glucuronide |
(B) Pelargonidin-3-galactoside |
(A) Antihyperglycmeic effect:
(B) Hypolipemic effect: |
Reduce hyperglycemia
Reduce the level of antioxidant defensive enzymes
Stimulate insulin secretion
Reduce the level of TBARS which is a byproduct of lipid peroxidation |
Streptozotocin (STZ)-induced diabetic rats
Diabetic rats |
|
[199][200][217,219] |
3. Challenges Using Flavonoids
3.1. Estimated Consumption Level of Flavonoids
3.1. Estimated Consumption Level of Flavonoids
Flavonoids derived from vegetables and fruits are consumed in low quantities. Moreover, the content of vegetables and fruits contain not only flavonoids, but also a mixture of secondary plant metabolites. Therefore, it is difficult to stimulate this mixture into a simple purified dietary supplement [201][202][220,221]. Efforts have been made to establish an optimal human dietary consumption level of flavonoids worldwide, but the estimate methods used were poorly established [203][222]. A U.S. study on 805 men aged 65–84 years reported that the estimate intake of flavonoids from quercetin, myricetin, kaempferol, apigenin, and leuteolin was 26 mg/d and the major sources of intake were in apples, tea, and onions [204][223]. Another study conducted in the Netherlands reported a two-times higher the level of flavonoids consumed in adults compared to the U.S. study (50 mg/day) [205][224]. In addition, two Dutch studies reported the estimated consumption level of flavonoids to be 23 mg/day and 26 mg/day respectively [206][207][225,226]. These differences observed in the consumption level of flavonoids depend on dietary habits, geographical location, socioeconomic status, food processing and preparation method, solubility of flavonoids, and the ethnicity of the population. For example, in Japan, soy containing food is highly consumed and, as a result the intake of isoflavone is higher than other flavonoids subclasses [110][106]. A study reported that orange juice contains 81–200mg/L of soluble flavanones compared to 206–644 mg/L seen in the cloud which clearly suggest that processing and storage affects the concentration of flavonoids [208][227].
3.1.1. Possible Side Eects of Flavonoids Consumption
Flavonoids in bacterial and mammalian experimental studies using Ames test indicated possible genotoxicity and mutagenicity of flavonoids if consumed at higher concentrations (ranges from 12.1 nmol to 225.0 nmol) [209]. Furthermore, it may alter amino acid, drug metabolism and the activity of key metabolizing enzymes [210]. Quercetin, a predominant flavonol in the human diet, showed a mutagenic effect by altering base-pair substitution and frame-shift mutation [211]. The isolated nuclei from liver rats treated with morin and naringenin showed an increase in reactive oxygen species, like hydroxyl radicals that lead to DNA degradation [212]. Additionally, flavonoids exert a cytotoxic activity as a topoisomerase II inhibitor. Genistein and quercetin are identified as topoisomerase II inhibitors, even at low concentrations (10 μM), where they accumulate cleavable complexes seen in patients with secondary leukemia [213]. Genistein, naringenin, kaempferol, and daidzein were reported to inhibit thyroxine synthesis by irreversibly inhibiting thyroid peroxidation [214]. Although no data are available to state the long-term side effects of increased flavonoid consumption, following an Asian diet that contains 68 mg of flavonol and 20–240 mg of isoflavone could improve thyroid function, reduce breast cancer mortality, and should not cause adverse health effects [215]. The concentrations needed for most flavonoids to generate mutagenic and cytotoxic side effects are unlikely to occur through dietary sources, but with supplementation, it could result in an increased toxic level. For instance, the recommended dosage of quercetin supplements is between 500 mg/day and 1000 mg/day, which is 20 times higher with what could be consumed in a vegetarian diet [216].Flavonoids in bacterial and mammalian experimental studies using Ames test indicated possible genotoxicity and mutagenicity of flavonoids if consumed at higher concentrations (ranges from12.1 nmol to 225.0 nmol) [228]. Furthermore, it may alter amino acid, drug metabolism and the activity of key metabolizing enzymes [229]. Quercetin, a predominant flavonol in the human diet, showed a mutagenic eect by altering base-pair substitution and frame-shift mutation [230]. The isolated nuclei from liver rats treated with morin and naringenin showed an increase in reactive oxygen species, like hydroxyl radicals that lead to DNA degradation [231]. Additionally, flavonoids exert a cytotoxic activity as a topoisomerase II inhibitor. Genistein and quercetin are identified as topoisomerase II inhibitors, even at low concentrations (10 M), where they accumulate cleavable complexes seen in patients with secondary leukemia [232]. Genistein, naringenin, kaempferol, and daidzein were reported to inhibit thyroxine synthesis by irreversibly inhibiting thyroid peroxidation [233]. Although no data are available to state the long-termside effects of increased flavonoid consumption, following an Asian diet that contains 68 mg of flavonol and 20–240 mg of isoflavone could improve thyroid function, reduce breast cancer mortality, and should not cause adverse health effects [234].The concentrations needed for most flavonoids to generate mutagenic and cytotoxic side effects are unlikely to occur through dietary sources, but with supplementation, it could result in an increased toxic level. For instance, the recommended dosage of quercetin supplements is between 500 mg/day and 1000 mg/day, which is 20 times higher with what could be consumed in a vegetarian diet [235].
3.1.2. Could Flavonoid Combinations have synergistic effects?
While the amounts of flavonoids consumed is crucial to establish positive effects but also to avoid negative effects, the tables list some flavonoids that trigger multiple selected pathways improving the pathogenesis of diabetes (Figure 3, Table 1, Table 2, Table 3 and Table 4). The better activity can be defined by the number of diabetes related pathways which are improved through the consumption of different flavonoids. The administration of baicalein triggers four pathways: The suppression in the NF-κB pathway and fatty acid synthesis; the activation in hexokinase activity in the liver; and the protection against cell apoptosis. Quercetin prompts the activity of three different pathways: It improves GLUT 4 translocation; inhibits tyrosine kinase activity; and reduces lipid peroxidation. β-cells apoptosis could be prevented by the administration of cyanidin or kaempferol, or baicalein. The consumption of rutin or cyanidin inhibits α-glucosidase and α-amylase which reduce carbohydrate absorption in the small intestine (Table 4).While the amounts of flavonoids consumed is crucial to establish positive eects but also to avoid negative effects, the tables list some flavonoids that trigger multiple selected pathways improving the pathogenesis of diabetes (Figure 3, Tables 1–4). The better activity can be defined by the number of diabetes related pathways which are improved through the consumption of different flavonoids.The administration of baicalein triggers four pathways: The suppression in the NF-B pathway and fatty acid synthesis; the activation in hexokinase activity in the liver; and the protection against cell apoptosis. Quercetin prompts the activity of three dierent pathways: It improves GLUT 4 translocation; inhibits tyrosine kinase activity; and reduces lipid peroxidation. -cells apoptosis could be prevented by the administration of cyanidin or kaempferol, or baicalein. The consumption of rutin or cyanidin inhibits -glucosidase and -amylase which reduce carbohydrate absorption in the small intestine (Table 4).
Could their positive effects on diabetes be further improved by ingesting a combination of different flavonoids which complement each other by triggering additional pathways? For example, the administration of baicalein and quercetin initiates the positive effects on diabetes in six major pathways: The glucose transporter; hepatic enzymes; beta cells apoptosis; PPARs; AMPK; tyrosine kinase; and NF-κB pathways. As a result of this hypothesized combination, the over activation of these pathways may be prevented, while the needed action to improve diabetes may be achieved. At this time, these are no more than suggestions which need to be proven by research. To date, little is known about flavonoids to flavonoids interactions [216]. In addition, some flavonoids showed an opposite effect on the same pathway and both lead to the improvement of diabetes. For example, fisetin has an inhibitory effect, while morin has a stimulatory effect on glucose 6 phosphate dehydrogenase and the literature states that they both improve diabetes (Figure 1). Extensive studies are required to understand the reasons behind this action—is it because of different binding sites, bioavailability, tissue exposure, absorption, or circulating concentration of these compounds. A similar pattern with different flavonoids was observed with PPAR and NF-κB pathways (Table 1, Table 2, Table 3 and Table 4).Could their positive effects on diabetes be further improved by ingesting a combination of different flavonoids which complement each other by triggering additional pathways? For example, the administration of baicalein and quercetin initiates the positive eects on diabetes in six major pathways: The glucose transporter; hepatic enzymes; beta cells apoptosis; PPARs; AMPK; tyrosine kinase; and NF-B pathways. As a result of this hypothesized combination, the over activation of these pathways may be prevented, while the needed action to improve diabetes may be achieved. At this time, these are no more than suggestions which need to be proven by research. To date, little is known about flavonoids to flavonoids interactions [235]. In addition, some flavonoids showed an opposite effects on the same pathway and both lead to the improvement of diabetes. For example, fisetin has an inhibitory effects, while morin has a stimulatory effects on glucose 6 phosphate dehydrogenase and the literature states that they both improve diabetes (Figure 1). Extensive studies are required to understand the reasons behind this action—is it because of different binding sites, bioavailability, tissue exposure, absorption, or circulating concentration of these compounds. A similar pattern with different flavonoids was observed with PPAR and NF-B pathways (Tables 1–4).