Isocitrate dehydrogenases (IDHs) are enzymes that catalyze the oxidative decarboxylation of isocitrate, producing
α
-ketoglutarate (
α
KG) and CO2. The discovery of IDH mutations in several malignancies has led to a better characterization of IDHs involvement in tumorigenesis and the approval of drugs targeting IDH1/2 mutants in cancers. Nevertheless, less is known about the impact of IDH mutants in rare pathologies or the relevance of non-mutated IDH enzymes in cancers. Here, we provide a brief overview of the impact of IDHs enzymes as potential therapeutic targets.
Table of contents
1. Isocitrate dehydrogenase enzymes
2. IDHs Genetic Alterations
3. Wild-type IDHs in Cancer
4. IDH Inhibition in Cancer Enhances Responsiveness to Canonical Therapies
[This is a summary basedu on the review paper by Bergaggio E, Piva R, 2019]
1. Isocitiorate Dehydrogenase en
zymes
Isocitrate dehydrogenases (IDHs) are enzymes that catalyze the oxidative decarboxylation of isocitrate to α-ketoglutarate (αKG), within the tricarboxylic acid (TCA) cycle. In humans, IDHs exist in three isoforms: IDH1, IDH2, and IDH3. Beyond their role in intermediary metabolism and energy production[1][2] [1,2], IDH enzymes - which use either NAD+ or NADP+ as cofactors- are involved also in redox status regulation in a plethora of cellular functions[3] [3]. Furthermore, αKG enables the activity of αKG-dependent dioxygenases required for essential processes, such as DNA and histone demethylation or DNA repair[4][5] [4,5].
2. IDHs Genetic Alterations
IDHs enzymes have recently become of particular interest as mutations of IDH1 and IDH2 genes have been found in several malignancies and genetic diseases, such as gliomas[6] [8], myeloid neoplasms[7] [9], Ollier disease and Maffucci syndrome[8] [10]. IDH1/2 mutant proteins display a new enzymatic activity able to catalyze the NADPH-dependent reduction of αKG, producing the oncometabolite D-2-hydroxyglutarate (D-2HG). This has a critical impact on the epigenetic cell status blocking cellular differentiation by competitively inhibiting αKG-dependent dioxygenases involved in histone and DNA demethylation, together with additional alterations in cellular metabolism, redox state and DNA repair[9][10] [11,12]. These discoveries prompted the development of IDH1/2-mutant inhibitors such as enasidenib (AG-221), and ivosidenib (AG-120), approved by the FDA for the treatment of refractory or relapsed acute myeloid leukemia mutated in IDH2 or IDH1, respectively[11][12] [13,14].
3. Wild-type IDHs in Cancer
Beyond IDHs mutations, aberrant expression of IDHs genes has also been related to carcinogenesis but its significance and their possible therapeutic implications remain to be further investigated. At present, it is not clear whether IDH1/2/3 activities may have a tumorigenic or tumor-suppressive role. While low expression of IDH1, IDH2, and IDH3 has been reported in several malignancies[13][14] [15,16], their overexpression has been found to promote tumor growth and therapy resistance in other cancers[15][16][17] [17
-19]. Overall, it emerges that the impact of IDH1/2/3 expression levels is strongly dependent on tumor type.
4. IDH Inhibition in Cancer Enhances Responsiveness to Canonical Therapies
Several lines of evidence suggest that IDH1/2/3 expression levels can be used as biomarkers for diagnosis, prognosis prediction, and target of therapy in specific neoplastic diseases. Additional studies also indicate that IDH1/2 inhibition could increase the efficacy of conventional cancer therapies, such as chemotherapy, radiotherapy, photodynamic therapy, and small molecule inhibitors[15][18][19] [17,20
,21]
. The combination significantly decreases tricarboxylic acid cycle activity and ATP levels which exacerbate the damage caused by canonical therapies increasing cell death (Figure 1). Importantly, this combinatorial effect occurs not only when IDH has an altered expression, but also when they are expressed at normal levels, increasing the applicability of IDH as potential targets for anticancer therapy. Since inhibitors directed against wild-type IDHs are not present on the market, further pre-clinical experiments could bring to the application of new drugs for IDH1/2 wild-type patients refractory to conventional therapy.
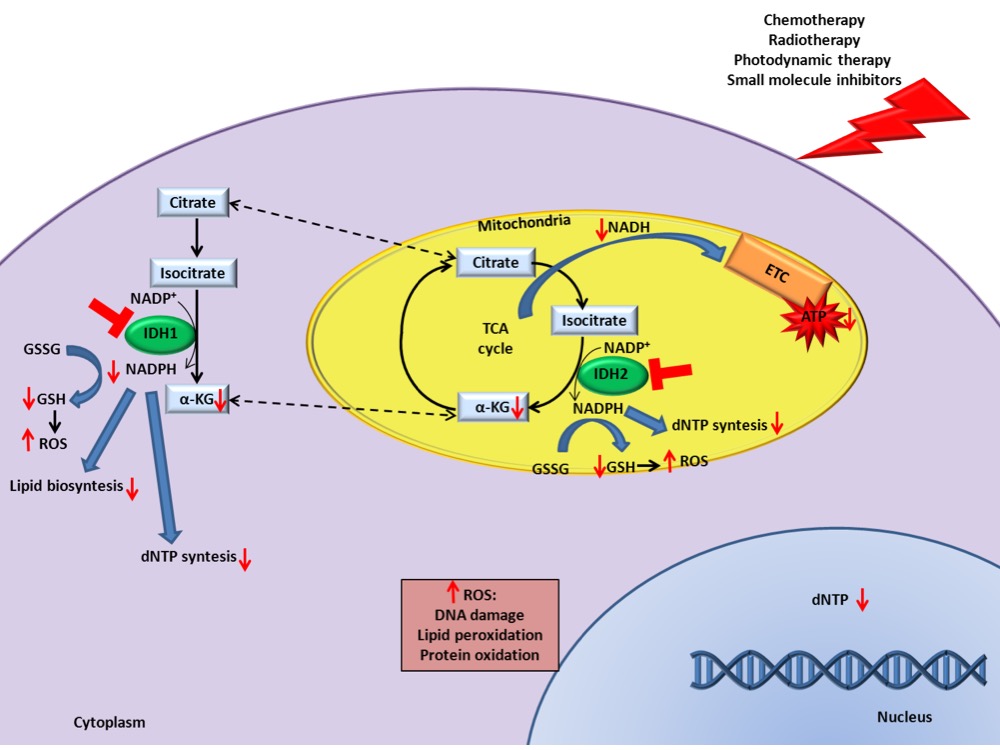
Figure 1. IDH inhibition enhances canonical cancer therapies efficacy. Schematic representation of the underlying mechanisms that increase the response to cancer therapies in presence of IDH1/2 inhibition.
References
- Wise, D.R.; Ward, P.S.; Shay, J.E.S.; Cross, J.R.; Gruber, J.J.; Sachdeva, U.M.; Platt, J.M.; DeMatteo, R.G.; Simon, M.C.; Thompson, C.B. Hypoxia promotes isocitrate dehydrogenase-dependent carboxylation of α-ketoglutarate to citrate to support cell growth and viability. Natl. Acad. Sci. U. S. A. 2011, 108, 19611–6.
- Mullen, A.R.; Wheaton, W.W.; Jin, E.S.; Chen, P.-H.; Sullivan, L.B.; Cheng, T.; Yang, Y.; Linehan, W.M.; Chandel, N.S.; DeBerardinis, R.J. Reductive carboxylation supports growth in tumour cells with defective mitochondria. Nature 2012, 481, 385–388.
- Ying, W. NAD+/NADH and NADP+/NADPH in Cellular Functions and Cell Death: Regulation and Biological Consequences. Antioxid. Redox Signal. 2008, 10, 179–206.
- Loenarz, C.; Schofield, C.J. Expanding chemical biology of 2-oxoglutarate oxygenases. Nat. Chem. Biol. 2008, 4, 152–156.
- Jochmanova, I.; Pacak, K. Pheochromocytoma: The First Metabolic Endocrine Cancer. Clin. Cancer Res. 2016, 22, 5001–5011.
- Yan, H.; Parsons, D.W.; Jin, G.; McLendon, R.; Rasheed, B.A.; Yuan, W.; Kos, I.; Batinic-Haberle, I.; Jones, S.; Riggins, G.J.; et al. IDH1 and IDH2 mutations in gliomas. N. Engl. J. Med. 2009, 360, 765–773.
- Balss, J.; Meyer, J.; Mueller, W.; Korshunov, A.; Hartmann, C.; von Deimling, A. Analysis of the IDH1 codon 132 mutation in brain tumors. Acta Neuropathol. 2008, 116, 597–602.
- Yan, H.; Parsons, D.W.; Jin, G.; McLendon, R.; Rasheed, B.A.; Yuan, W.; Kos, I.; Batinic-Haberle, I.; Jones, S.; Riggins, G.J.; et al. IDH1 and IDH2 mutations in gliomas. N. Engl. J. Med. 2009, 360, 765–773
- Molenaar, R.J.; Thota, S.; Nagata, Y.; Patel, B.; Clemente, M.; Przychodzen, B.; Hirsh, C.; Viny, A.D.; Hosano, N.; Bleeker, F.E.; et al. Clinical and biological implications of ancestral and non-ancestral IDH1 and IDH2 mutations in myeloid neoplasms. Leukemia 2015, 29, 2134–2142.
- Pansuriya, T.C.; van Eijk, R.; d’Adamo, P.; van Ruler, M.A.J.H.; Kuijjer, M.L.; Oosting, J.; Cleton-Jansen, A.-M.; van Oosterwijk, J.G.; Verbeke, S.L.J.; Meijer, D.; et al. Somatic mosaic IDH1 and IDH2 mutations are associated with enchondroma and spindle cell hemangioma in Ollier disease and Maffucci syndrome. Nat. Genet. 2011, 43, 1256–1261.
- Gagné, L.M.; Boulay, K.; Topisirovic, I.; Huot, M.-É.; Mallette, F.A. Oncogenic Activities of IDH1/2 Mutations: From Epigenetics to Cellular Signaling. Trends Cell Biol. 2017, 27, 738–752.
- Lu, C.; Ward, P.S.; Kapoor, G.S.; Rohle, D.; Turcan, S.; Abdel-Wahab, O.; Edwards, C.R.; Khanin, R.;
Figueroa, M.E.; Melnick, A.; et al. IDH mutation impairs histone demethylation and results in a block to cell differentiation. Nature 2012, 483, 474–478
- Kim, E.S. Enasidenib: First Global Approval. Drugs 2017, 77, 1705–1711.
- DiNardo, C.D. Ivosidenib in IDH1 -Mutated Acute Myeloid Leukemia. N. Engl. J. Med. 2018, 379, 1186.
- Robbins, D.; Wittwer, J.A.; Codarin, S.; Circu, M.L.; Aw, T.Y.; Huang, T.-T.; Van Remmen, H.; Richardson, A.; Wang, D.B.; Witt, S.N.; et al. Isocitrate dehydrogenase 1 is downregulated during early skin tumorigenesis which can be inhibited by overexpression of manganese superoxide dismutase. Cancer Sci. 2012, 103, 1429–1433.
- Tian, G.-Y.; Zang, S.-F.; Wang, L.; Luo, Y.; Shi, J.-P.; Lou, G.-Q. Isocitrate Dehydrogenase 2 Suppresses the Invasion of Hepatocellular Carcinoma Cells via Matrix Metalloproteinase 9. Cell. Physiol. Biochem. 2015, 37, 2405–2414
- Calvert, A.E.; Chalastanis, A.; Wu, Y.; Hurley, L.A.; Kouri, F.M.; Bi, Y.; Kachman, M.; May, J.L.; Bartom, E.; Hua, Y.; et al. Cancer-Associated IDH1 Promotes Growth and Resistance to Targeted Therapies in the Absence of Mutation. Cell Rep. 2017, 19, 1858–1873.
- Li, J.; He, Y.; Tan, Z.; Lu, J.; Li, L.; Song, X.; Shi, F.; Xie, L.; You, S.; Luo, X.; et al. Wild-type IDH2 promotes the Warburg effect and tumor growth through HIF1α in lung cancer. Theranostics 2018, 8, 4050–4061.
- Zeng, L.; Morinibu, A.; Kobayashi, M.; Zhu, Y.; Wang, X.; Goto, Y.; Yeom, C.J.; Zhao, T.; Hirota, K.; Shinomiya, K.; et al. Aberrant IDH3α expression promotes malignant tumor growth by inducing HIF-1-mediated metabolic reprogramming and angiogenesis. Oncogene 2015, 34, 4758–4766.
- Li, S.; Chou, A.P.; Chen, W.; Chen, R.; Deng, Y.; Phillips, H.S.; Selfridge, J.; Zurayk, M.; Lou, J.J.; Everson, R.G.; et al. Overexpression of isocitrate dehydrogenase mutant proteins renders glioma cells more sensitive to radiation. Neuro-Oncology 2013,15,57–68.
- Bergaggio, E.; Riganti, C.; Garaffo, G.; Vitale, N.; Mereu, E.; Bandini, C.; Pellegrino, E.; Pullano, V.; Omedè, P.; Todoerti, K.; et al. IDH2 inhibition enhances proteasome inhibitor responsiveness in hematological malignancies. Blood 2019, 133, 156–167.