Note:All the information in this draft can be edited by authors. And the entry will be online only after authors edit and submit it.
1. Introduction
Nitrogen-containing heterocyclic compounds play an important role in the drug discovery process, as approximately 75% of FDA (Food and Drug Administration)-approved small-molecule drugs contain one or more nitrogen-based heterocycles [1]. Thiazole, or 1,3-thiazole, belongs to the class of azoles and contains one sulfur atom and one nitrogen atom at positions 1 and 3. Its diverse biological activity is reflected in a large number of clinically approved thiazole-containing compounds with an extensive range of pharmacological activities. Most of these compounds are 2,4-disubstituted thiazole derivatives, and only a few are 2,5-disubstituted or 2,4,5-trisubstituted thiazoles [2].
Several drugs such as sulfathiazole; aztreonam; numerous cephems (ceftaroline, cefotiam, ceftibuten, cefixime, ceftriaxone, cefotaxime, ceftazidime, cefmenoxime, ceftizoxime, cefepime, cefdinir) with antibacterial effects; pramipexole with antiparkinsonian activity; edoxaban with antithrombotic effects; isavuconazole with antifungal effects; famotidine and nizatidine with antiulcer activity; meloxicam with anti-inflammatory effects; tiazofurin, dabrafenib, dasatinib, ixabepilone, and epothilone with antitumor effects; mirabegron as a β3-adrenergic receptor agonist; nitazoxanide and thiabendazole with antiparasitic effects; and febuxostat with antigout activity contain one thiazole moiety in the structure (Figure 1) [2–4].
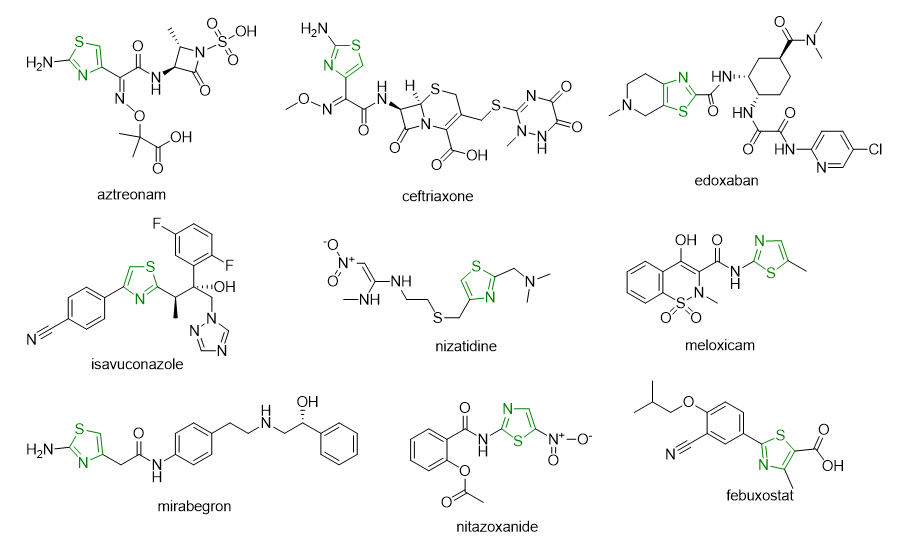
Figure 1. Clinical drugs bearing one thiazole ring.
Compounds bearing two thiazole rings, such as the antitumor drug bleomycin, the antiretroviral agent ritonavir, the pharmacokinetic enhancer for HIV drugs cobicistat, and the antibacterial agent cefditoren, have been authorized on the pharmaceutical market (Figure 2) [2,5].
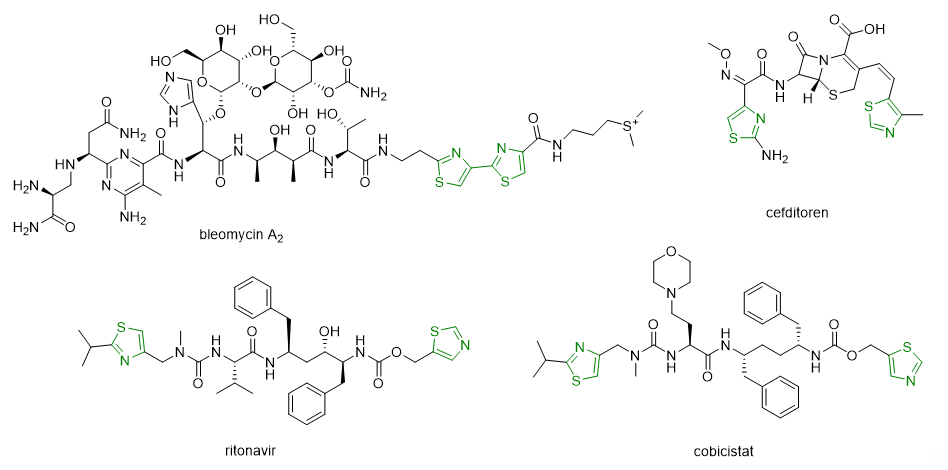
Figure 2. Clinical drugs bearing two thiazole rings.
The high medicinal significance of this scaffold has attracted considerable attention from many researchers and encouraged the design and synthesis of numerous thiazole- and bisthiazole-containing compounds with diverse pharmacological activities, such as antibacterial [6], antifungal [7], antiprotozoal [8], antiviral [9,10], anticancer [11], anti-inflammatory [12–16], antioxidant [17], analgesic [18], anticonvulsant [19], antidiabetic [20], and antihypertensive [21] activities. Furthermore, thiazole compounds exhibiting a promising biological potential for the treatment of Alzheimer’s disease [22,23] and metabolic syndrome [24] have been reported in the scientific literature.
2. Chemistry of Thiazole
Free thiazole is a pale-yellow flammable liquid, with a pyridine-like odor and a boiling point in the range of 116-118 °C. It has an aromatic character, due to the delocalization of a lone pair of electrons from the sulfur atom, resulting in a 6π-electron system. Also, its high aromaticity is highlighted by proton nuclear magnetic resonance, the chemical shift values of each proton within the thiazole ring being situated between 7.27 and 8.77 ppm. The resonance structures of thiazole are illustrated in Figure 3 [25,26].

Figure 3. The resonance structures of thiazole.
The calculated π-electron density revealed that the electrophilic substitution takes place preferentially at the C-5 position, followed by the C4-position (Figure 4). The nucleophilic substitution occurs at the C-2 position [27].
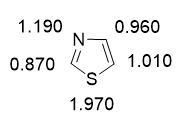
Figure 4. Calculated π-electron density of thiazole.
The acidity given by the presence of the three hydrogen atoms decreases in the order H2 >> H5 > H4 [4].
3. Synthesis of Thiazole and Bisthiazole Derivatives
Hantzsch synthesis is the oldest and most widely known method for the synthesis of a thiazole ring. The method consists of a cyclization reaction between alpha-halocarbonyl compounds and various reactants containing the N-C-S fragment. Examples of such compounds include thiourea, thioamides, thiosemicarbazides, and thiosemicarbazones [28].
The condensation of thioamides with various alpha-halocarbonyl compounds is commonly used. Many thiazoles with alkyl, aryl, or heteroaryl substituents at position 2, 4, or 5 can be obtained through this reaction. The reaction mechanism consists of the nucleophilic attack of the thioamide sulfur atom on the alpha carbon of the alpha-halocarbonyl, with the formation of an intermediate compound, which by subsequent dehydration leads to the corresponding thiazole (Scheme 1).
By the reaction of thiourea with alpha-halocarbonyl compounds, monosubstituted or disubstituted 2-aminothiazoles can be obtained, while by using other compounds containing thioamide moieties, such as thiosemicarbazides and thiosemicarbazones, 2-hydrazinothiazole and thiazol-2-yl-hydrazone derivatives can be synthesized in good yields. The condensation reactions occur through imino thioether and hydroxythiazoline intermediates, which are sometimes stable and isolable. The alpha-halocarbonyl component may be represented by alpha-haloketones and alpha-haloesters [29,30].

Scheme 1. Reaction mechanism of Hantzsch thiazole synthesis.
Moreover, thiazoles can be obtained through Gabriel synthesis [25,31], Cook-Heilbron synthesis [29,30], or by reacting various compounds such as isocyanide derivatives and carboxymethyl dithioates [32], propargyl bromides and thiourea derivatives [33], oximes, anhydrides and potassium thiocyanate [34], aldehydes, amines and element sulfur [35], vinyl azides and potassium thiocyanate [36].
Numerous heterocyclic compounds possessing the thiazole ring have been obtained in good to excellent yields using microwave irradiation [37,38]. Compared to conventional methods, microwave-assisted synthesis has several advantages, being an environmentally friendly and cost-effective tool, that leads to improved yields in short reaction times [39].
The synthesis of symmetrical 2,2`-bisthiazole compounds occurs most frequently by condensation of dithiooxamide with α-bromoketones at a ratio of 1:2, in ethanol at reflux [40-42].
The synthesis of symmetrical 4,4`-bisthiazole derivatives is most often performed by a condensation reaction of thioamides with 1,4-dibromo-2,3-butanedione, in a molar ratio of 2:1, in ethanol at reflux [43,44].
Several methods for the synthesis of 5,5`-bisthiazole compounds, starting from halogenated derivatives by transition metal-catalyzed arylation or cross-coupling reactions, have been reported in the literature [45,46].
Numerous protocols for the synthesis of thiazolyl-linker-thiazole compounds have been reported in the literature, most of them being based on the Hantzsch condensation reaction. The reaction can occur in a single step, when the two thiazole rings are formed simultaneously, using starting compounds such as bis-thiosemicarbazones, 2,5-dithiobiurea, bis-hydrazonoyl halides or dihalo diketones, or in two steps respectively, when the two thiazole rings are formed successively [47–52].
References
- Kerru, N.; Gummidi, L.; Maddila, S.; Gangu, K.K.; Jonnalagadda, S.B. A Review on Recent Advances in Nitrogen-Containing Molecules and Their Biological Applications. Molecules 2020, 25, 1909, doi:10.3390/molecules25081909.
- Scott, K.A.; Njardarson, J.T. Analysis of US FDA-Approved Drugs Containing Sulfur Atoms. Curr. Chem. 2018, 376, 5, doi:10.1007/s41061-018-0184-5.
- Sharma, P.C.; Bansal, K.K.; Sharma, A.; Sharma, D.; Deep, A. Thiazole-containing compounds as therapeutic targets for cancer therapy. J. Med. Chem. 2020, 188, 112016, doi:10.1016/j.ejmech.2019.112016.
- Pola, S. Significance of Thiazole-based Heterocycles for Bioactive Systems. In Scope of Selective Heterocycles from Organic and Pharmaceutical Perspective; Varala, R., Ed.; InTech: Rijeka, Croatia, 2016; pp. 1–47.
- Gallant, J.E.; Koenig, E.; Andrade-Villanueva, J.; Chetchotisakd, P.; DeJesus, E.; Antunes, F.; Arastéh, K.; Moyle, G.; Rizzardini, G.; Fehr, J.; et al. Cobicistat Versus Ritonavir as a Pharmacoenhancer of Atazanavir Plus Emtricitabine/Tenofovir Disoproxil Fumarate in Treatment-Naive HIV Type 1–Infected Patients: Week 48 Results. Infect. Dis. 2013, 208, 32–39, doi:10.1093/infdis/jit122.
- Mishra, I.; Mishra, R.; Mujwar, S.; Chandra, P.; Sachan, N. A retrospect on antimicrobial potential of thiazole scaffold. Heterocycl. Chem. 2020, 57, 2304–2329, doi:10.1002/jhet.3970.
- Meleddu, R.; Distinto, S.; Corona, A.; Maccioni, E.; Arridu, A.; Melis, C.; Bianco, G.; Matyus, P.; Cottiglia, F.; Sanna, A.; et al. Exploring the thiazole scaffold for the identification of new agents for the treatment of fluconazole resistant Candida. Enz. Inhib. Med. Chem. 2016, 31, 1672–1677, doi:10.3109/14756366.2015.1113171.
- da Silva, E.B.; Oliveira e Silva, D.A.; Oliveira, A.R.; da Silva Mendes, C.H.; dos Santos, T.A.R.; da Silva, A.C.; de Castro, M.C.A.; Ferreira, R.S.; Moreira, D.R.M.; Cardoso, M.V. de O.; et al. Desing and synthesis of potent anti-Trypanosoma cruzi agents new thiazoles derivatives which induce apoptotic parasite death. J. Med. Chem. 2017, 130, 39–50, doi:10.1016/j.ejmech.2017.02.026.
- Singh, I.P.; Gupta, S.; Kumar, S. Thiazole Compounds as Antiviral Agents: An Update. Chem. (Los. Angeles). 2020, 16, 4–23, doi:10.2174/1573406415666190614101253.
- Abu-Melha, S.; Edrees, M.M.; Riyadh, S.M.; Abdelaziz, M.R.; Elfiky, A.A.; Gomha, S.M. Clean grinding technique: A facile synthesis and in silico antiviral activity of hydrazones, pyrazoles, and pyrazines bearing thiazole moiety against SARS-CoV-2 main protease (Mpro). Molecules 2020, 25, doi:10.3390/molecules25194565.
- Gomha, S.; Edrees, M.; Altalbawy, F. Synthesis and Characterization of Some New Bis-Pyrazolyl-Thiazoles Incorporating the Thiophene Moiety as Potent Anti-Tumor Agents. J. Mol. Sci. 2016, 17, 1499, doi:10.3390/ijms17091499.
- Woods, K.W.; McCroskey, R.W.; Michaelides, M.R.; Wada, C.K.; Hulkower, K.I.; Bell, R.L. Thiazole analogues of the NSAID indomethacin as selective COX-2 Inhibitors. Med. Chem. Lett. 2001, 11, 1325–1328, doi:10.1016/S0960-894X(01)00212-8.
- Araniciu, C.; Pârvu, A.; Palage, M.; Oniga, S.; Benedec, D.; Oniga, I.; Oniga, O. The Effect of Some 4,2 and 5,2 Bisthiazole Derivatives on Nitro-Oxidative Stress and Phagocytosis in Acute Experimental Inflammation. Molecules 2014, 19, 9240–9256, doi:10.3390/molecules19079240.
- Liaras, K.; Fesatidou, M.; Geronikaki, A. Thiazoles and Thiazolidinones as COX/LOX Inhibitors. Molecules 2018, 23, 685, doi:10.3390/molecules23030685.
- Abdelall, E.K.A.; Kamel, G.M. Synthesis of new thiazolo-celecoxib analogues as dual cyclooxygenase-2/15-lipoxygenase inhibitors: Determination of regio-specific different pyrazole cyclization by 2D NMR. J. Med. Chem. 2016, 118, 250–258, doi:10.1016/j.ejmech.2016.04.049.
- Rödl, C.B.; Vogt, D.; Kretschmer, S.B.M.; Ihlefeld, K.; Barzen, S.; Brüggerhoff, A.; Achenbach, J.; Proschak, E.; Steinhilber, D.; Stark, H.; et al. Multi-dimensional target profiling of N,4-diaryl-1,3-thiazole-2-amines as potent inhibitors of eicosanoid metabolism. J. Med. Chem. 2014, 84, 302–311, doi:10.1016/j.ejmech.2014.07.025.
- Jaishree, V.; Ramdas, N.; Sachin, J.; Ramesh, B. In vitro antioxidant properties of new thiazole derivatives. Saudi Chem. Soc. 2012, 16, 371–376, doi:10.1016/j.jscs.2011.02.007.
- Saravanan, G.; Alagarsamy, V.; Prakash, C.R.; Kumar, P.D.; Selvam, T.P. Synthesis of Novel Thiazole Derivatives as Analgesic Agents. Asian J. Pharm. Sci. 2011, 1, 134–138.
- Siddiqui, N.; Ahsan, W. Triazole incorporated thiazoles as a new class of anticonvulsants: Design, synthesis and in vivo screening. J. Med. Chem. 2010, 45, 1536–1543, doi:10.1016/j.ejmech.2009.12.062.
- Wang, G.; Peng, Z.; Gong, Z.; Li, Y. Synthesis, biological evaluation, and docking studies of novel 5,6-diaryl-1,2,4-triazine thiazole derivatives as a new class of α-glucosidase inhibitors. Chem. 2018, 78, 195–200, doi:10.1016/j.bioorg.2018.03.015.
- Sowjanya, C.; Seetaram Swamy, S.; Gomathi, S.; Ashok Babu, K.; Synthesis, Chemistry and Anti-Hypertensive Activity of Some New Thiazole-Thiadiazole Derivatives. J. Adv. Res. Med. Pharm. Sci. 2016, 1, 6–10.
- Sahin, Z.; Ertas, M.; Bender, C.; Bülbül, E.F.; Berk, B.; Biltekin, S.N.; Yurttaş, L.; Demirayak, Ş. Thiazole-substituted benzoylpiperazine derivatives as acetylcholinesterase inhibitors. Drug Dev. Res. 2018, 79, 406–425, doi:10.1002/ddr.21481.
- Sagar, S.R.; Singh, D.P.; Das, R.D.; Panchal, N.B.; Sudarsanam, V.; Nivsarkar, M.; Vasu, K.K. Pharmacological investigation of quinoxaline-bisthiazoles as multitarget-directed ligands for the treatment of Alzheimer’s disease. Chem. 2019, 89, 1–17, doi:10.1016/j.bioorg.2019.102992.
- Kadam, K.S.; Jadhav, R.D.; Kandre, S.; Guha, T.; Reddy, M.M.K.; Brahma, M.K.; Deshmukh, N.J.; Dixit, A.; Doshi, L.; Srinivasan, S.; et al. Evaluation of thiazole containing biaryl analogs as diacylglycerol acyltransferase 1 (DGAT1) inhibitors. J. Med. Chem. 2013, 65, 337–347, doi:10.1016/j.ejmech.2013.05.006.
- Ayati, A.; Emami, S.; Asadipour, A.; Shafiee, A.; Foroumadi, A. Recent applications of 1,3-thiazole core structure in the identification of new lead compounds and drug discovery. J. Med. Chem. 2015, 97, 699–718, doi:10.1016/j.ejmech.2015.04.015.
- Sarangi, P.K.N.; Sahoo, J.; Swain, B.D.; Paidesetty, S.K.; Mohanta, G.P. Thiazoles as potent anticancer agents: A review. Indian Drugs 2016, 53, 5–11.
- Eicher, T.; Hauptmann, S. The Chemistry of Heterocycles. Structure, Reactions, Syntheses, and Applications; WILEY-VCH GmbH & Co. KGaA: Weinheim, Germany, 2003; ISBN 3-527-30720-6.
- Leoni, A.; Locatelli, A.; Morigi, R.; Rambaldi, M. Novel thiazole derivatives: A patent review (2008-2012; Part 1). Expert Opin. Ther. Pat. 2014, 24, 201–216, doi:10.1517/13543776.2014.858121.
- Mishra, C.B.; Kumari, S.; Tiwari, M. Thiazole: A promising heterocycle for the development of potent CNS active agents. J. Med. Chem. 2015, 92, 1–34, doi:10.1016/j.ejmech.2014.12.031.
- Ali, S.H.; Sayed, A.R. Review of the synthesis and biological activity of thiazoles. Commun. 2020, 50, 1–31, doi:10.1080/00397911.2020.1854787.
- Nayak, S.; Gaonkar, S.L. A Review on Recent Synthetic Strategies and Pharmacological Importance of 1,3-Thiazole Derivatives. Mini-Reviews Med. Chem. 2019, 19, 215–238, doi:10.2174/1389557518666180816112151.
- Lingaraju, G.; Swaroop, T.; Vinayaka, A.; Sharath Kumar, K.; Sadashiva, M.; Rangappa, K. An Easy Access to 4,5-Disubstituted Thiazoles via Base-Induced Click Reaction of Active Methylene Isocyanides with Methyl Dithiocarboxylates. Synthesis (Stuttg). 2012, 44, 1373–1379, doi:10.1055/s-0031-1290762.
- Castagnolo, D.; Pagano, M.; Bernardini, M.; Botta, M. Domino Alkylation-Cyclization Reaction of Propargyl Bromides with Thioureas/Thiopyrimidinones: A New Facile Synthesis of 2-Aminothiazoles and 5H-Thiazolo[3,2-a]pyrimidin-5-ones. Synlett 2009, 2009, 2093–2096, doi:10.1055/s-0029-1217700.
- Tang, X.; Yang, J.; Zhu, Z.; Zheng, M.; Wu, W.; Jiang, H. Access to Thiazole via Copper-Catalyzed [3+1+1]-Type Condensation Reaction under Redox-Neutral Conditions. Org. Chem. 2016, 81, 11461–11466, doi:10.1021/acs.joc.6b02124.
- Wang, X.; Qiu, X.; Wei, J.; Liu, J.; Song, S.; Wang, W.; Jiao, N. Cu-Catalyzed Aerobic Oxidative Sulfuration/Annulation Approach to Thiazoles via Multiple Csp 3 –H Bond Cleavage. Lett. 2018, 20, 2632–2636, doi:10.1021/acs.orglett.8b00840.
- Chen, B.; Guo, S.; Guo, X.; Zhang, G.; Yu, Y. Selective Access to 4-Substituted 2-Aminothiazoles and 4-Substituted 5-Thiocyano-2-aminothiazoles from Vinyl Azides and Potassium Thiocyanate Switched by Palladium and Iron Catalysts. Lett. 2015, 17, 4698–4701, doi:10.1021/acs.orglett.5b02152.
- Gomha, S.M.; Edrees, M.M.; Faty, R.A.M.; Muhammad, Z.A.; Mabkhot, Y.N. Microwave-assisted one pot three-component synthesis of some novel pyrazole scaffolds as potent anticancer agents. Cent. J. 2017, 11, 37, doi:10.1186/s13065-017-0266-4.
- Karamthulla, S.; Pal, S.; Khan, M.N.; Choudhury, L.H. “On-water” synthesis of novel trisubstituted 1,3-thiazoles via microwave-assisted catalyst-free domino reactions. RSC Adv. 2014, 4, 37889–37899, doi:10.1039/C4RA06239F.
- Prajapati, N.P.; Patel, K.D.; Vekariya, R.H.; Patel, H.D.; Rajani, D.P. Thiazole fused thiosemicarbazones: Microwave-assisted synthesis, biological evaluation and molecular docking study. Mol. Struct. 2019, 1179, 401–410, doi:10.1016/j.molstruc.2018.11.025.
- Lai, L.-F.; Ho, C.-L.; Chen, Y.-C.; Wu, W.-J.; Dai, F.-R.; Chui, C.-H.; Huang, S.-P.; Guo, K.-P.; Lin, J.-T.; Tian, H.; et al. New bithiazole-functionalized organic photosensitizers for dye-sensitized solar cells. Pigment. 2013, 96, 516–524, doi:10.1016/j.dyepig.2012.10.002.
- Liu, L.; Lam, Y.-W.; Wong, W.-Y. Complexation of 4,4′-di(tert-butyl)-5-ethynyl-2,2′-bithiazole with mercury(II) ion: Synthesis, structures and analytical applications. Organomet. Chem. 2006, 691, 1092–1100, doi:10.1016/j.jorganchem.2005.11.014.
- Cao, J.; Curtis, M.D. Synthesis and characterizations of oligomeric (4,4′-dialkyl-2,2′-bithiazole) carboxylic acids and their LB-film behavior. Met. 2005, 148, 219–226, doi:10.1016/j.synthmet.2004.09.031.
- Khavasi, H.R.; Abedi, A.; Amani, V.; Notash, B.; Safari, N. Synthesis, characterization and crystal structure determination of zinc (II) and mercury (II) complexes with 2,2′-dimethyl-4,4′-bithiazole. Polyhedron 2008, 27, 1848–1854, doi:10.1016/j.poly.2008.02.029.
- Sasaki, H. Synthesis of 2,2′-Bis(3,6,9-triazanonyl)-4,4′-bithiazole and Related Compounds as New DNA Cleavage Agents. Pharm. Bull. (Tokyo). 2007, 55, 1762–1767, doi:10.1248/cpb.55.1762.
- Turner, G.L.; Morris, J.A.; Greaney, M.F. Direct Arylation of Thiazoles on Water. Chemie Int. Ed. 2007, 46, 7996–8000, doi:10.1002/anie.200702141.
- Stanetty, P.; Schnürch, M.; Mihovilovic, M.D. Halogenated 2‘-Chlorobithiazoles via Pd-Catalyzed Cross-Coupling Reactions. Org. Chem. 2006, 71, 3754–3761, doi:10.1021/jo0601009.
- Rajanarendar, E.; Ramakrishna, S.; Rama Murthy, K. Synthesis of novel isoxazolyl bis-thiazolo[3,2-a]pyrimidines. Chinese Chem. Lett. 2012, 23, 899–902, doi:10.1016/j.cclet.2012.06.029.
- Hosny, M.; Salem, M.E.; Darweesh, A.F.; Elwahy, A.H.M. Synthesis of Novel Bis(thiazolylchromen-2-one) Derivatives Linked to Alkyl Spacer via Phenoxy Group. Heterocycl. Chem. 2018, 55, 2342–2348, doi:10.1002/jhet.3296.
- Shawali, A.S. A review on bis-hydrazonoyl halides: Recent advances in their synthesis and their diverse synthetic applications leading to bis-heterocycles of biological interest. Adv. Res. 2016, 7, 873–907, doi:10.1016/j.jare.2016.09.001.
- Borcea, A.-M.; Marc, G.; Pîrnău, A.; Vlase, L.; Ionuț, I.; Tiperciuc, B.; Oniga, O. Synthesis and molecular docking study of some new 1,4-phenylene-bisthiazoles as fungal lanosterol 14α-demethylase inhibitors. Farmacia 2017, 65, 683–689.
- Hassan, A.A.; El-Sheref, E.M. Chemistry and heterocyclization of dithiobiurea and thioureidoalkylthiourea. Heterocycl. Chem. 2010, 47, 764–784, doi:10.1002/jhet.406.
- M. Gomha, S.; M. Abdelrazek, F.; H. Abdelrahman, A.; Metz, P. Synthesis of Some Novel Thiazole, Thiadiazole and 1,4-Phenylene-bis-thiazole Derivatives as Potent Antitumor Agents. Heterocycles 2016, 92, 954, doi:10.3987/COM-16-13443.