Colorectal cancer (CRC) is one of the most common causes of death and the third most diagnosed cancer worldwide, one of the main risk for developing CRC is a diet high in fat and carbohydrates which stimulates an inflammatory state increasing reactive oxygen species (ROS). Dietary Bioactive compounds have antioxidant proprieties and participates in gut microbiota modulation, decreasing reactive oxygen species and inflammation, both principal causes of cancer. These compounds can promote apoptosis and inhibit cell growth, proliferation, and migration of colorectal cancer cells modulating molecular pathways related to CRC. The multidisciplinary tratment of CRC requires a Nutritional intervention since patient nutritional status affects treatment response and the progression or recurrence of the tumor
1. Introduction
Colorectal cancer (CRC) is third in incidence worldwide and second in mortality. One of the main risk factors for developing CRC is a lifestyle with poor physical activity and a diet high in fat and carbohydrates, which stimulates an inflammatory state that increases reactive oxygen species (ROS) and releases proinflammatory cytokines in the intestinal mucosa
[1]. Nutritional status influences part of the response to treatment in cancer patients. For example, malnutrition predicts worse results in response to treatment and survival
[2]; also, recurrence, progression, and the presence of metastasis have been attributed to poor nutritional status
[3].
The bioactive compounds of food have been of interest since they may contribute to maintaining human health. These compounds are present in small amounts in plants and certain foods such as fruits, vegetables, nuts, and seeds. They can interact with tissue components, potentially promoting multiple health benefits
[4]. Bioactive compounds have a wide spectrum of chemical structures, and their main function is as antioxidants; they also modulate the expression and activity of transcription growth factors and inflammatory mediators and are cell cycle intermediaries
[1][5][1,5]. They can modulate immunoregulator molecules such as PD-L1 by modulating mitochondria activity and hypoxia, ubiquitination, glycosylation, and endoplasmic reticulum degradation, among others
[6][7][8][9][6,7,8,9]. It has been proposed to consider the bioavailability of the compound rather than the amount present in food or dietary supplements to determine the benefit of the compound. It has been reported that the DNA damage repair mechanism can activate the expression of PD-L1 since BRCA2 or Ku70/80 depletion enhances PD-L1 upregulation after DSBs in an ATM/ATR/Chk1-dependent manner
[10][11][12][10,11,12]. The consumption of various bioactive compounds, such as phenolic compounds, flavonoids, phenolic acids, carotenoids, and xanthophylls, influences the preservation of cell integrity, the maintenance of cell DNA repair systems, and their action in cell differentiation, proliferation, and apoptosis.
2. Generalities of Bioactive Compounds in CRC
Bioactive products have always played a significant role as novel therapeutical agents, irrespective of their source of origin. They are phenolic compounds, flavonoids, phenolic acids, carotenoids, and xanthophylls. Different concentrations are found in vegetables, fruits, grains, spices, and their derived foods and beverages, such as tea, olive oil, fruit juices, wine, chocolate, and beer, as shown in
Table 1.
Table 1.
Bioactive compounds and food sources.
Natural Products
|
Bioactive Compound
|
Food Sources
|
Flavonoids
|
Curcumin
|
Turmeric, Ginger, Curry
|
|
Resveratrol
|
Red wine, Red grapes, Peanuts
|
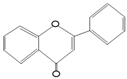
|
Quercetin
|
Onions, Tea, Apples, Kale
|
|
Epigallocatechin Gallate
|
Green tea, White tea, Black tea
|
|
Anthocyanins
|
Blackberries, Raspberries, Cherries
|
Phenolic Acids
|
Caffeic Acid
|
Coffee beans, olives, potatoes, carrots, propolis
|
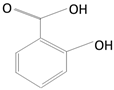
|
Ellagic Acid
|
Pomegranates, blackberries, raspberries, strawberries
|
|
Gallic Acid
|
Grapes, strawberries, blueberries, mango, plums, hazelnut
|
Carotenoids
|
α-carotenoid
|
Carrots, sweet potatoes, pumpkin, broccoli,
spinach
|

|
β-carotenoid
|
Carrots, sweet potatoes, pumpkin, spinach, kale
|
|
Lycopene
|
Tomatoes, watermelon, grapefruits
|
Xanthophylls
|
β-cryptoxanthin
|
Citrus fruits, papaya, egg yolk, apples
|
|
Astaxanthin
|
Seafood, tomato
|
|
Fucoxanthin
|
Brown seaweeds
|
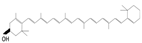
|
Lutein
|
Broccoli, spinach, kale, kiwi, grapes, pumpkin
|
|
Zeaxanthin
|
Broccoli, spinach, kale, orange, peppers
|
3. Regulation of Molecular Pathways in Colorectal Carcinogenesis by Bioactive Compounds
A distinct hallmark of cancer is metabolism pathways, which is why research is focused on knowing how to regulate the metabolism pathways related to CRC, potentially using this knowledge to improve current treatments. The Wnt pathway, best known as Wnt/B catenin, participates in cancer initiation and progression and regulates the pluripotency of cells during development and differentiation
[13][45]. The invasion and prognosis of metastasis are related to the epithelial–mesenchymal transition (EMT), the malignant proliferation of tumor cells, decreased apoptosis, and the regulation of expression of EMT markers. A loss of E-cadherin expression is a molecular key point
[14][46]. Another molecular mechanism for developing CRC is the signal transducer and activator of transcription (STAT). In this regard, seven STAT factors share molecular characteristics that control their action mode; specifically, STAT1 and STAT3 have an important role in CRC progression
[15][47]. Moreover, cytokines such as IFN-γ, IL-6, IL-1, and EGF
[16][48] activate a family of proteins associated with their receptors, the Janus kinases (JAK), which activate STAT molecules. The most studied in CRC is JAK2/STAT3 since they relate to EMT. The JAK/STAT pathway promotes invasion, migration, growth, and chemoresistance
[17][49].
The tumor microenvironment is the different cell types surrounding the tumor, including fibroblasts, endothelial cells, macrophages, dendritic cells, tumor stem cells, adipocytes, and the microbiome. They are key in promoting or restricting tumor development
[18][19][20][50,51,52]. The changes that occur in the tumor microenvironment resemble a state of chronic inflammation, which begins with ischemia that continues to interstitial edema, the infiltration of immune cells, and angiogenesis, suggesting that the tumors are infiltrated by inflammatory cells and cytokines PDGF, EGF, IL-1, TNFα, and TGFβ
[21][53] that favor oxidative stress and the presence of ROS
[22][54].
CRC begins with altering the regulatory mechanisms of DNA repair systems and the cell proliferation of mucosa cells lining the colon and rectum. These cells convert into neoplasia that develops polyps that advance to high-grade dysplasia and evolve into invasive tumors. Early genetic mutations include the BRAF and APC genes
[23][55].
CRC develops due to cancer stem cells (CSC), pluripotent stem cells with self-renewal capacity to different lineages found in the colon, promoting carcinogenesis and favoring tumor heterogeneity
[24][56]. Microbiota and metabolome alterations are involved in pro- or anti-cancer actions
[25][57]. Several phytochemicals involved in regulating the Hedgehog, Notch, and Wnt/β-catenin pathways, such as curcumin, quercetin, lycopene, cinnamic acid, resveratrol, sibylline, and EGCG, have been identified. Their importance in regulating pathways as they regulate the maintenance and proliferation of CSCs is summarized below. The inflammation process promotes cancer progression through inflammatory cytokines in the tumor microenvironment, such as TNF-α, which is a mediator involved in chronic inflammatory diseases with greater participation during the early stages of carcinogenesis, angiogenesis, invasion, generating reactive oxygen, and nitrogen species
[26][27][58,59]. Also, cytokines such as IL-2 and IL-6 favor tumor proliferation, inhibit apoptosis, and participate in the conversion of non-cancerous cells to tumor stem cells. Moreover, TGF-b improves invasion and metastasis by inducing the transition epithelium-mesenchymal cells and IFN-γ participate in metastasis
[28][60]. Lastly, Wnt signaling is involved with NF-ĸB and MAPK; together, they can increase oxidative stress and inhibit apoptosis
[29][30][61,62].
Curcumin and quercetin are the most studied bioactive compounds regarding CRC. On the one hand, curcumin is a natural and active derivative of turmeric. It is an oily molecule soluble in acetic acid and ketones but appears insoluble in water. It possesses anti-angiogenic, anti-tumor, antiproliferative, and anti-inflammatory properties through various mechanisms, including the suppression of intrinsic and extrinsic apoptotic signaling pathways, cell cycle arrest, and the activation of autophagy. Curcumin, obtained from the dried root of Curcuma longa, can kill only tumor cells without harming healthy cells due to a high expression of a protein known as GADD45a (the gene activated during DNA damage). However, there are limitations in its use as a therapeutic agent since it has low solubility, poor absorption, rapid metabolism, and rapid elimination, which is why the use of nanoparticles has been implemented to encapsulate it so that it can achieve its therapeutic effect within the tumor
[31][32][89,90] On the other hand, Quercetin is a secondary metabolite of plants. It has an anti-tumor effect by regulating the signal transduction pathways to prevent, inhibit or reverse carcinogenesis. It also has anti-inflammatory and antioxidant effects. It may potentiate cytotoxic effects or reduce the side effects of chemotherapeutic drugs on normal cells or reverse drug susceptibility
[32][90].
Numerous health benefits of these food supplements are recognized by pioneering experimental studies involving both in vitro and in vivo studies in recent decades, including their antioxidant and anti-inflammatory potential, digestive stimulant effects, hypolipidemic actions, antilithogenic properties, anti-diabetic influence, antimutagenic, and anticancer potential
[33][91]. Studies have shown that spices and their bioactive compounds can inhibit or even activate the pathways related to cell division, proliferation, and detoxification, in addition to having immunomodulatory and anti-inflammatory effects
[34][92].
4. Chemosensitive and Chemopreventive Properties by Bioactive Compounds in CRC
There are several applications for polyphenols and carotenoids that have not been sufficiently explored, such as their potential use for predisposition to CRC
[35][36][93,94], sensitizers to current CRC standard chemotherapy
[37][38][23,39] candidates for treatment
[39][40][95,96], and the reduction in its recurrence
[41][97]. Using polyphenols and carotenoids as sensitizers and buffers to chemotherapy and radiotherapy could decrease drug resistance and minimize toxicity by requiring lower doses
[42][43][98,99]. Further research into the adjuvant treatment of CRC with dietary polyphenols and carotenoids may yield improved outcomes for patients with lower costs and minimal risks.
These bioactive compounds have recently been found to protect against chemotherapy side effects or modify susceptibility by reversing chemoresistance (
Figure 1). For example, curcumin attenuates oxaliplatin-induced liver injury by activating nuclear erythroid factor 2-related factor 2 (Nrf2) signaling, regulating cellular defense pathways, and the oxidative damage to mitochondria caused by oxaliplatin
[44][100]. Furthermore, curcumin protects against irinotecan-induced intestinal injury inhibiting NF-ĸB activation
[45][101]. It is also active against FOLFIRI and bevacizumab cardiotoxicity by suppressing oxidative stress and preventing mitochondrial dysfunction in cardiac mitochondria
[46][102]. Regarding quercetin, reports from specific cell lines (SW620) exposed to this bioactive compound appeared to be more sensitive to doxorubicin due to the inhibition of ATP-driven transport activity of P-glycoprotein that leads to a higher concentration of doxorubicin at the intracellular level. Also, it has been proposed that quercetin can reverse multidrug resistance by regulating the expression of glutamine transporter 1 member 5 (SLC1A5)
[47][103].
Figure 1.
Bioactive compounds and their participation in decreasing CRC.
The studies of resveratrol against cancer have demonstrated chemopreventive functions related to anti-inflammatory, antioxidant, anti-apoptosis, and anti-proliferative properties
[48][49][50][51][104,105,106,107]. Resveratrol may reduce the side effects of chemotherapy, such as renal toxicity, cardiotoxicity, gastrointestinal toxicity, hepatotoxicity, and UVR-induced skin cancer
[52][108]. In addition, resveratrol has been used as a chemosensitizer in CRC cells (HCT116, HT-29, and SW620) to 5-FU by inducing cell cycle arrest and apoptosis independently of p53 status and inhibiting their endogenous antioxidant capacity, respectively
[53][54][109,110]. In a study with etoposide-resistant HT-29 cells, resveratrol was a chemosensitizer, inducing cell cycle inhibition, ROS formation, AMPK activation, and apoptosis induction
[55][111]. The activation of AMPK and SIRT1 has long been thought to be the mechanism via which dietary bioactive compounds influence their health benefits. According to Liu et al., resveratrol inhibits proliferation and induces death in ovarian cancer cells (A2780 and SKOV3) by reducing glycolysis and targeting the AMPK/mTOR signaling pathway
[56][112]. In breast cancer, resveratrol regulates EMT by modulating TGF-β1. Furthermore, resveratrol can induce autophagy by upregulating SIRT3 and phosphorylated AMPK, suggesting that the resveratrol-mediated inhibition of tumor progression is attributed to the participation of the SIRT3/AMPK/autophagy signaling axis
[57][113]. Another example is curcumin-induced AMPK and its downstream factor ACC phosphorylation in lung cancer cells
[58][59][114,115] and prostate cancer
[60][116]. EGCG promotes cell survival by shifting the balance of the mTOR-AMPK pathway during ER stress
[61][117]. The indirect AMPK activators show promise in treating various disorders such as cancer. The effects of systemic and chronic AMPK activation remain unknown.
Resveratrol also sensitizes CRC cells to 5-FU by inhibiting EMT factors (vimentin and SNAI2 proteins), increasing intercellular junctions (desmosomes, gap, and tight junctions, and adhesion molecules such as E-cadherin), and inhibiting the NF-kB pathway. Also, it has been reported that this compound downregulates p-AKT in cancer cell lines; however, it also upregulates the same pathway to prevent paclitaxel-induced neuropathic pain or ischemia-reperfusion injuries
[62][118]. The combined treatment of 5-FU and resveratrol in colorectal cancer sensitizes tumor cells to 5-FU, which induces a further increase in oxidative stress related to the inhibition of the AKT and STAT3 proteins
[63][119] known for their oncogenic potential in colorectal carcinomas
[64][120].When resveratrol is combined with forskolin, it has phosphodiesterase 4D inhibitory effects to inhibit AKT/mTOR signaling in colorectal cancer cells
[65][121]. In the same polyphenol family, EGCG can decrease the probability of recurrence of colorectal adenocarcinoma
[66][122] in combination with cisplatin or oxaliplatin, evidenced by an improved therapeutic effect.
Another chemosensitizer mechanism to 5-FU by resveratrol is through miR-34 regulation. This mechanism was studied in vitro using HCT116 cell lines. The mechanism was mediated by inhibiting the PI3K/Akt and MAPK Erk1/2 signaling pathways that increased miR-34a production and thus indirectly suppressed the
SIRT1 gene via
E2F3 gene expression
[67][68][123,124]. Also, a study found that resveratrol sensitizes HT-29 and HCT-116 CRC cells to oxaliplatin, increasing the expression of miR-34c, which inhibits its target, KITLG
[69][125]. Moreover, when resveratrol and oxaliplatin are combined, the cell proliferation of Caco-2 CRC cells decreases due to the induction of apoptosis and necrosis
[70][126].
According to studies, cinnamic acid has cardioprotective
[71][128] and hepatoprotective activity and an analgesic effect during chemotherapy
[72][129]. For example, an in vivo model with rats (Sprague Dawley) treated with oxaliplatin developed side effects; meanwhile, when cinnamic acid was provided, neuropathic pain decreased
[73][130].
There are a few studies of cinnamic acid and CRC as a chemosensitizer; however, it has been recently demonstrated that cinnamic acid alone or combined with FOLFOX reduces side population cells and cancer stem cell markers in the HT29 cell line
[74][73]. However, the chemosensitizer potential of cinnamic acid in chemotherapy has not been explored, so
thwe
researchers cconsider it a promising candidate for study.
Curcumin can reverse chemoresistance by upregulating EMT markers by attenuating the TGF-β/Smad2/3 signaling pathway or downregulating TET1-NKD2-WNT
[75][131]. Curcumin as an adjuvant treatment to FOLFOX chemotherapy in CRC patients did not decrease neurotoxicity or quality of life but improved overall survival in the curcumin group versus those with only chemotherapy
[76][132]. However, in vitro curcumin has been able to sensitize colon cancer stem cells to chemotherapy drugs such as 5-FU, FOLFOX, and irinotecan, further reducing the emergence of chemoresistant cells
[77][70]. In addition, curcumin and resveratrol have been linked to the ability to prevent breast metastasis and gastric cancer, osteosarcoma, and lung cancer through the coordinated action of several molecular pathways such as the SIRT3/AMPK/autophagy signal axis, JAK2/STAT3, and MALAT1/miR-383-5p/DDIT4
[57][113]. Quercetin involves reduced metastasis by negatively regulating the expression of the protein survivin, which is expressed in the macrophages that regulate the processes of proliferation and cell death. This bioactive compound also regulates cyclin D1, which controls the cell cycle.