In the last decade, nanotechnology allowed for an improvement of materials properties in many application fields, such as sensing [1–9], optoelectronics [10–15], energy [16–21], catalysis [22–24] and biotechnology [25–31]. Gold was used to produce many different nanostructures through a bottom-up approach, such as spheres, rods, stars, cubes, hollow nanoparticles, and nanocapsules, widely used in biomedicine and reported in a schematic way in Figure 1. These nanostructures showed remarkable chemical-physical properties, and often their superficial functionalizations allow a response to environmental changes, such as variations in the temperature, pH, light, and redox potentials [6,8,26,30,32–35]. This represented an amazing opportunity for their use in active therapies, as drug carriers, as theranostic agents, and as sensing materials [6,26,30,33,36–39]. In particular, localized surface plasmon resonance (LSPR) plays an important role in several nanotechnological applications. Electrons on the surface of noble metal nanoparticles, interacting with electromagnetic radiation, generate LSPR and, for this reason, metal nanoparticles produce strong extinction and scattering spectra, useful for many and different applications.
Other application examples are the visualization methods that use gold nanoparticles and confocal laser microscopy, which show great attractiveness in the biomedical and biosensing field [40]. In fact, several methods, such as fluorescence detection (confocal fluorescence microscopy) or resonance elastic or two-photon light scattering (resonance scattering confocal microscopy or two-photon luminescence confocal microscopy) resulted in notable confocal images [41,42]. The main advantage of this method is the background signal decrease and, at the same time, the enhancement of the contrast. Another popular method in biological imaging is represented by dark-field microscopy, in which the objects with a size under the resolution limit of a light microscope, induce light scattering. A new application was developed by American researchers at the El Sayed laboratory, based on the spherical gold nanoparticles (AuNPs) preferentially bonded to cancerous cells, as compared with binding to healthy cells, using AuNPs conjugated with antibodies specific to tumor antigens [43]. In these cases, resonance scattering dark-field microscopy is used to map a tumor with a high accuracy. In successive studies, gold nanorods, nanostars, and nanocages were used with the same aim [44,45]. Among others, some modern methods for biological imaging have recently been developed; they can be called biophotonic methods, and they study the light-biological matter interaction. Optical coherence tomography, X-ray and magneto-resonance tomography, photoacoustic microscopy and tomography, as well as fluorescence correlation microscopy, can be included as part of the biophotonic methods [46].
Among others, Raman imaging of surface enhanced Raman scattering (SERS) nanoparticles is an optical technique that offers an incomparable sensitivity (on the order of 10−15–10−12 M) and multiplexing abilities to the field of molecular imaging. Raman spectroscopy is due to the inelastic scattering of light upon interaction with a molecule, used to produce a sample fingerprint. Raman scattering has an inherently weak effect. However, if the incident photon loses or gains energy as it interacts with the molecule, this produces Stokes or anti-Stokes Raman scattering, respectively. SERS consists of the interaction of a Raman reporter with a roughened metal surface, which gives an electromagnetic enhancement of signal of the order of 104 to 108 over spontaneous Raman. It can be exploited for sensing and for diagnoses. The SERS technique is used to realize innovative probes that associate metallic nanoparticles with specific organic Raman reporter molecules. These SERS-active probes are used to indirectly sense the target molecules by using laser Raman spectrometry or SERS microscopy. Therefore, they show optical labeling functions like organic ones. These kinds of probes have a typical ultrasensitivity, as well as the multiplexing and quantitative skills of the SERS technique, and they show amazing potentials for bioanalysis [6,26,30].
Gold-based materials, with various dimensions and shapes, are also used in these methods, both for therapeutic and diagnostic applications [47]. Furthermore, several studies have been conducted to verify their low toxicity. Generally the results show how the toxicity and cytotoxicity of gold nanomaterials depend on the size and surface chemistry: they are mostly nontoxic after acute exposures, as long as the particles are around 4–5 nm in diameter [48–50], while particles larger than 5 nm can have toxic effects due to toxic surface coatings [51,52]. Often, acute toxicity can be attributed to the use of very high concentrations or specific cell type sensitivities [53,54].
In a wide panorama of innovative materials, this review tries to give an up-to-date view of the progress of nanosized gold-based materials. In fact, the last years have seen the creation of numerous morphologies, schematically summarized in six shapes in Figure 1, such as spheres (AuNPs), rods (AuNRs), stars (AuNSs), cubes (AuNCs), hollow particles (AuHNPs), and capsules and cages (AuNCaps and AuNcages), each one with advantages and critical issues. Moreover, the importance of surface specific chemistry has a crucial role in view of biomedical applications, and the multidisciplinary research activities take this point greatly into account.
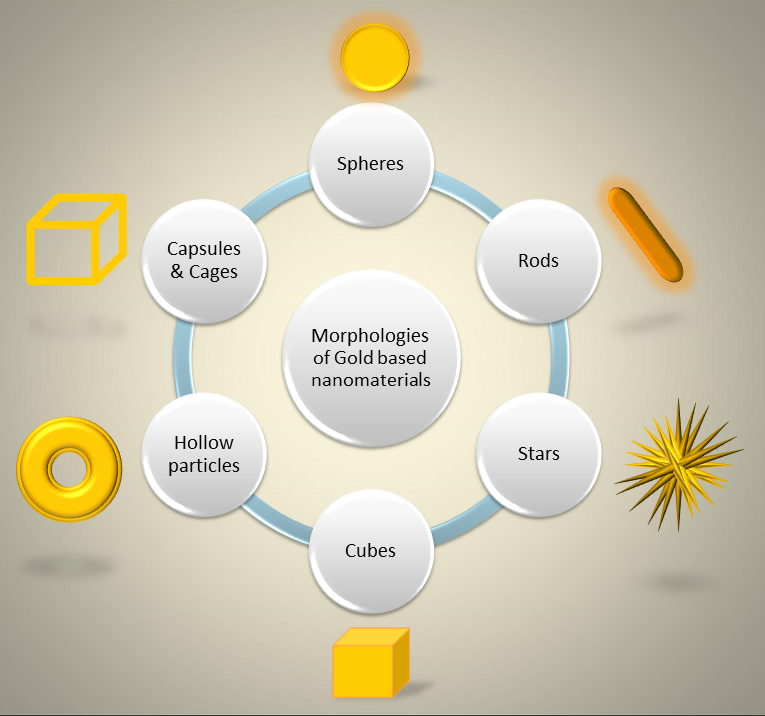
Figure 1. Schematic representation of the main morphologies of gold-based nanomaterials used in biomedical applications.
References
- Li, N.; Zhao, P.; Astruc, D. Anisotropic gold nanoparticles: Synthesis, properties, applications, and toxicity. Chem. Int. Ed. Engl. 2014, 53, 1756–1789.
- Ma, Y.; Zhu, Y.; Liu, B.; Quan, G.; Cui, L. Colorimetric Determination of Hypochlorite Based on the Oxidative Leaching of Gold Nanorods. Materials 2018, 11, 1629.
- Pantalei, S.; Zampetti, E.; Macagnano, A.; Bearzotti, A.; Venditti, I.; Russo, M.V. Enhanced sensory properties of a multichannel quartz crystal microbalance coated with polymeric nanobeads. Sensors 2007, 7, 2920–2928.
- Jiang, D.F.; Pang, J.; You, Q.N.; Liu, T.; Chu, Z.Y.; Jin, W.Q. Simultaneous biosensing of catechol and hydroquinone via a truncated cube-shaped Au/PBA nanocomposite. Bioelectron. 2019, 124, 260–267.
- Venditti, I.; Fratoddi, I.; Bearzotti, A. Self-assembled copolymeric nanoparticles as chemically interactive materials for humidity sensors. Nanotechnology 2010, 21, 8.
- Wang, Y.; Yan, B.; Chen, L. SERS tags: Novel optical nanoprobes for bioanalysis. Rev. 2013, 113, 1391–1428.
- D’Amato, R.; Venditti, I.; Russo, M.V.; Falconieri, M. Growth Control and Long range Self-assembly of Polymethylmethacrylate Nanospheres. Appl. Polymer Sci. 2006, 102(5), 4493–4499.
- Luo, C.D.; Wang, Y.Y.; Li, X.M.; Jiang, X.Q.; Gao, P.P.; Sun, K.; Zhou, J.H.; Zhang, Z.G.; Jiang, Q. An Optical Sensor with Polyaniline-Gold Hybrid Nanostructures for Monitoring pH in Saliva. Nanomaterials 2017, 7(3), 11.
- Ciotta, E.; Paoloni, S.; Richetta, M.; Prosposito, P.; Tagliatesta, P.; Lorecchio, C.; Venditti, I.; Fratoddi, I.; Casciardi, S.; Pizzoferrato, R. Sensitivity to Heavy-Metal Ions of Unfolded Fullerene Quantum Dots. Sensors 2017, 17(11), 15.
- Chen, Z.; Choi, C.K.K.; Wang, Q. Origin of the Plasmonic Chirality of Gold Nanorod Trimers Templated by DNA Origami. ACS Appl Mater Inter. 2018, 10(32), 26835–26840.
- Matassa, R.; Familiari, G.; Battaglione, E.; Sibilia, C.; Leahu, G.; Belardini, A.; Venditti, I.; Fontana, L.; Fratoddi, I. Electron microscopy reveals a soluble hybrid network of individual nanocrystals self-anchored by bifunctional thiol fluorescent bridges. Nanoscale 2016, 8(42), 18161–18169.
- Zhang, W.; Caldarola, M.; Lu, X.; Pradhan, B.; Orrit, M. Single-molecule fluorescence enhancement of a near-infrared dye by gold nanorods using DNA transient binding. Chem. Chem. Phys. 2018, 20(31), 20468–20475.
- Venditti, I.; D’Amato, R.; Russo, M.V.; Falconieri, M. Synthesis of conjugated polymeric nanobeads for photonic bandgap materials. Actuat. B-Chem. 2007, 126(1), 35–40.
- Vestler, D.; Shishkin, I.; Gurvitz, E.A.; Nasir, M.E.; Ben-Moshe, A.; Slobozhanyuk, A.P.; Krasavin, A.V.; Levi-Belenkova, T.; Shalin, A.S.; Ginzburg, P.; et al. Circular dichroism enhancement in plasmonic nanorod metamaterials. Express 2018, 26(14), 17841–17848.
- De Angelis, R.; Venditti, I.; Fratoddi, I.; De Matteis, F.; Prosposito, P.; Cacciotti, I.; D’Amico, L.; Nanni, F.; Yadav, A.; Casalboni, M.; et al. From nanospheres to microribbons: Self-assembled Eosin Y doped PMMA nanoparticles as photonic crystals. Colloid Interf. Sci. 2014, 414, 24–32.
- Razi, M.; Wang, R.; He, Y.Y.; Kirby, R.M.; Dal Negro, L. Optimization of Large-Scale Vogel Spiral Arrays of Plasmonic Nanoparticles. Plasmonics 2019, 14(1), 253–261.
- Mousli, F.; Chaouchi, A.; Hocine, S.; Lamouri, A.; Vilar, M.R.; Kadri, A.; Chehimi, M.M. Diazonium-modified TiO2/polyaniline core/shell nanoparticles. Structural characterization, interfacial aspects and photocatalytic performances. Surf. Sci. 2019, 465, 1078–1095.
- Naponiello, G.; Venditti, I.; Zardetto, V.; Saccone, D.; Di Carlo, A.; Fratoddi, I.; Barolo, C.; Dini, D. Photoelectrochemical characterization of squaraine-sensitized nickel oxide cathodes deposited via screen-printing for p-type dye-sensitized solar cells. Surf. Sci. 2015, 356, 911–920.
- Chang, S.; Li, Q.; Xiao, X.; Wong, K.Y.; Chen, T. Enhancement of low energy sunlight harvesting in dye-sensitized solar cells using plasmonic gold nanorods. Environ. Sci. 2012, 5, 9444–9448.
- Ali, A.K.; Erten-Ela, S.; Hassoon, K.I.; Ela, C. Plasmonic enhancement as selective scattering of gold nanoparticles based dye sensitized solar cells. Thin Solid Films 2019, 671, 127–132.
- Bonomo, M.; Naponiello, G.; Venditti, I.; Zardetto, V.; Di Carlo, A.; Dini, D. Electrochemical and Photoelectrochemical Properties of Screen-Printed Nickel Oxide Thin Films Obtained from Precursor Pastes with Different Compositions. Electrochem. Soc. 2017, 164(2), H137–H147.
- Chong, S.; Yang, T.C.K. Parametric Studies of Titania-Supported Gold-Catalyzed Oxidation of Carbon Monoxide. Materials 2017, 10(7), 12.
- Zhao, X.H.; Li, Z.C.; Deng, Y.J.; Zhao, Z.H.; Li, X.W.; Xia, Y.Z. Facile Synthesis of Gold Nanoparticles with Alginate and Its Catalytic Activity for Reduction of 4-Nitrophenol and H2O2 Materials 2017, 10(5), 11.
- Kim, I.; Seo, M.G.; Choi, C.; Kim, J.S.; Jung, E.; Hang, G.H.; Lee, J.C.; Han, S.S.; Ahn, J.P.; Jung, Y.; et al. Studies on Catalytic Activity of Hydrogen Peroxide Generation according to Au Shell Thickness of Pd/Au Nanocubes. ACS Appl. Mater. Inter. 2018, 10(44), 38109–38116.
- Porcaro, F.; Carlini, L.; Ugolini, A.; Visaggio, D.; Visca, P.; Fratoddi, I.; Venditti, I.; Meneghini, C.; Simonelli, L.; Marini, C.; et al. Synthesis and Structural Characterization of Silver Nanoparticles Stabilized with 3-Mercapto-1-Propansulfonate and 1-Thioglucose Mixed Thiols for Antibacterial Applications. Materials 2016, 9(12), 15.
- Fasolato, C.; Giantulli, S.; Silvestri, I.; Mazzarda, F.; Toumia, Y.; Ripanti, F.; Mura, F.; Luongo, F.; Costantini, F.; Bordi, F.; et al. Folate-based single cell screening using surface enhanced Raman microimaging. Nanoscale 2016, 8, 17304–17313.
- Prosposito, P.; Mochi, F.; Ciotta, E.; Casalboni, M.; De Matteis, F.; Venditti, I.; Fontana, L.; Testa, G.; Fratoddi, I. Hydrophilic silver nanoparticles with tunable optical properties: Application for the detection of heavy metals in water. Beilstein J. Nanotechnol. 2016, 7, 1654–1661.
- Moon, H.J.; Ku, M.; Lee, H.; Yoon, N.; Yang, J.; Bong, K.W. Implantable Photothermal Agents based on Gold Nanorods-Encapsulated Microcube. Rep. 2018, 8(1), 13683.
- Venditti, I. Morphologies and functionalities of polymeric nanocarriers as chemical tools for drug delivery: A review. King Saud Univ. Sci. 2017, doi.:10.1016/j.jksus.2017.10.004.
- Bassi, B.; Dacarro, G.; Galinetto, P.; Giulotto, E.; Marchesi, N.; Pallavicini, P.; Pascale, A.; Perversi, S.; Taglietti, A. Tailored coating of gold nanostars: Rational approach to prototype of theranostic device based on SERS and photothermal effects at ultralow irradiance. Nanotechnology 2018, 8, 235301.
- Placido, T.; Tognaccini, L.; Howes, B.D.; Montrone, A.; Laquintana, V.; Comparelli, R.; Curri, M.L.; Smulevich, G.; Agostiano, A. Surface Engineering of Gold Nanorods for Cytochrome. ACS Omega 2018, 3(5), 4959–4967.
- Hu, R.; Zheng, M.X.; Wu, J.C.; Li, C.; Shen, D.Q.; Yang, D.; Li, L.; Ge, M.F.; Chang, Z.M.; Dong, W.F. Core-Shell Magnetic Gold Nanoparticles for Magnetic Field-Enhanced Radio-Photothermal Therapy in Cervical Cancer. Nanomaterials 2017, 7(5), 11.
- Lee, J.H.; Lee, T.; Choi, J.W. Nano-Biosensor for Monitoring the Neural Differentiation of Stem Cells. Nanomaterials 2016, 6(12), 11.
- Cheheltani, R.; Ezzibdeh, R.M.; Chhour, P.; Pulaparthi, K.; Kim, J.; Jurcova, M.; Hsu, J.C.; Blundell, C.; Litt, H.I.; Ferrari, V.A.; et al. Tunable, biodegradable gold nanoparticles as contrast agents for computed tomography and photoacoustic imaging. Biomaterials 2016, 102, 87–97.
- Venditti, I. Gold Nanoparticles in Photonic Crystals Applications: A Review. Materials 2017, 10(2), 18.