Sex maintenance in mammals is important in sexual differentiation. The crucial event in mammalian sexual differentiation occurs at the embryonic stage of sex determination, when the bipotential gonads differentiate as either testes or ovaries, according to the sex chromosome constitution of the embryo, XY or XX, respectively. Once differentiated, testes produce sexual hormones that induce the subsequent differentiation of the male reproductive tract. On the other hand, the lack of masculinizing hormones in XX embryos permits the formation of the female reproductive tract. It was long assumed that once the gonad is differentiated, this developmental decision is irreversible. However, several findings in the last decade have shown that this is not the case and that a continuous sex maintenance is needed. Deletion of Foxl2 in the adult ovary lead to ovary-to-testis transdifferentiation and deletion of either Dmrt1 or Sox9/Sox8 in the adult testis induces the opposite process. In both cases, mutant gonads were genetically reprogrammed, showing that both the male program in ovaries and the female program in testes must be actively repressed throughout the individual’s life. In addition to these transcription factors, other genes and molecular pathways have also been shown to be involved in this antagonism.
1. Introduction
The assumption that gonadal differentiation is irreversible was motivated by the fact that most cases of XX or XY sex reversal could be explained by functional failure of sex-specific factors at the sex determination stage, suggesting that alterations were never produced at later stages. However, cases of transdifferentiation between gonadal sex-specific cell lines, mainly the supporting cell line, after sex determination have long been described. For instance, the ovary of old rats contained structures resembling testis cords that were not present in young rats
[1]. Additionally, several cases were reported in which testis-like structures developed in the ovary after the loss of the meiotic germ cells. E12.0 mouse ovaries transplanted beneath the kidney capsules of adult male mice initially developed as ovaries, but seminiferous cords with Sertoli-like cells and testosterone-producing Leydig cells started to develop from day 12 after transplantation in regions depleted of oocytes
[2]. Similarly, E14.5 rat ovaries cultured in a medium conditioned by either fetal or young testes, lost germ cells, and developed testis-like cords after 12 days of culture
[3], and undifferentiated tammar wallaby ovaries transplanted under the skin of young male pouch also became depleted of germ cells and contained seminiferous-like cords 25 days after transplantation
[4]. In addition, granulosa cells survive, proliferate, and subsequently acquire morphological characteristics of Sertoli cells in rat ovarian follicles depleted of oocytes by irradiation
[5]. We also showed that after depletion of germ cells in the medullary region of the developing XX gonad of the Iberian mole,
Talpa occidentalis, testis cord-like structures are formed and Leydig cells subsequently appear in the interstitial spaces
[6].
Another case of granulosa-to-Sertoli cell transdifferentiation was observed in freemartinism, a syndrome in which XX female cattle fetuses with male twins exhibit female-to-male sex reversal with varying degrees of female reproductive tract misdevelopment
[7]. In about 50% of the cases, the XX gonads present seminiferous-like tubules
[7], and in the most severe cases, Leydig-like cells were also described
[8]. Freemartinism occurs when chorionic vascular anastomosis allows the transfer of male gonad derived hormones to the female twin embryo, affecting its sexual development. Because the male reproductive ducts (Müllerian ducts) regress at the same time in the freemartin female as in her male twin, it was proposed that the Anti-Müllerian hormone (AMH, also known as Müllerian inhibiting substance, MIS), a member of the transforming growth factor β family produced by Sertoli cells that induces the regression of Müllerian ducts in male fetuses
[9], could be the factor responsible for the syndrome. Indeed,
in vitro culture of E14.5 rat ovaries in the presence of purified bovine, AMH showed a reduction of the gonadal volume, oocyte depletion, and differentiation of Sertoli-like cells
[10]. Likewise, transgenic mice chronically expressing
Amh presented ovaries with few germ cells at birth that were lost afterward, coinciding with the formation of seminiferous-like tubules
[9]. The molecular mechanism underlying this process remains unknown. It has been suggested that it is the loss of oocytes derived from the presence of AMH (this hormone is cytotoxic for oocytes), rather than the presence of AMH itself, which causes the transdifferentiation
[11][12]. However, this is controversial as the effect of oocyte depletion on granulosa cell transdifferentiation is not well understood. It seems to depend on the stage of germ cell development, and does not occur if the germ cells are depleted before the pre-meiotic stages
[13]. However, since postnatal oocyte depletion (like in ovaries exposed to AMH) leads to transdifferentiation in some cases
[5], but not in others
[14], it cannot be ruled out that AMH may have a direct role in the process of granulosa cell transdifferentiation.
Cell transdifferentiation has also been described in cases of human gonadal cancers. The Sertoli-Leydig cell tumor (SLCT) of the ovary is a rare type of tumor normally affecting middle-aged women, which is characterized by the presence of testicular structures including Sertoli-like cells and Leydig cells that produce androgens
[15]. On the other hand, cases of granulosa-cell tumors have also been reported in which neoplastic proliferation of intratubular sex cord cells progresses to an invasive tumor, simultaneously experiencing granulosa cell differentiation and losing Sertoli cell features
[16][17]. However, in both cases, supporting cell transdifferentiation may be just a secondary consequence of the dramatic alterations taking place in the genetic program of tumor cells.
Additional evidence of the plasticity of the gonadal cell fate came from transgenic mice.
Sry ectopic expression in XX embryonic gonads using a heat-shock-inducible system revealed that
Sox9 expression was upregulated and maintained in pre-granulosa cells when
Sry expression was induced during the E11.0–11.25 critical time window, but not afterward
[18]. However, fine monitoring of granulosa cells in these mice revealed that the SRY-dependent
Sox9 inducibility was not as transient in a subpopulation of pre-granulosa cells near the mesonephric tissue, which maintained that capability throughout fetal and early postnatal stages. Furthermore, when E13.5 ovaries were grafted into adult male nude mice, the heat-shock
Sry transgene was also able to induce
Sox9 expression in differentiated granulosa cells
[19].
Finally, chromatin accessibility landscape analyses using purified Sertoli cells have shown that this cell type maintains open chromatin regions near female-promoting genes that are enriched in transcription factor binding motifs for male-promoting genes such as
Sox9 and
Dmrt1, indicating that these genes are continuously acting as silencers by binding repressors of the granulosa cell fate
[20][21]. ChIP-seq for H3K27me3 and H3K4me3 provided results consistent with this notion. H3K27me3 indicates promoter repression, whereas H3K4me3 evidences promoter activation. ChIP-seq experiments performed using purified adult Sertoli cells showed that male-promoting genes harbored H3K4me3 and were depleted in H3K27me3, whereas female-promoting genes were enriched for both marks, indicating that female-determining genes persist in a poised state even long after Sertoli cell differentiation
[22]. These results indicate that cells of the supporting lineage require the opposite sex to be permanently repressed, and explain why the loss of such repressors can lead to transdifferentiation into the opposite cell lineage even long after the sex determination stage.
Cases of XX and XY sex reversal induced after sex determination have been associated with a number of sex-specific genes and pathways. We will review the most outstanding cases as follows.
2. Antagonism between Male and Female Factors in Sexual Cell Fate Maintenance
Sex determination involves not only the activation of genes necessary for the differentiation of a sexual fate, but also the active repression of the genetic program of the opposite sex
[23][24][25][26][27][28][29]. Some of these molecular interactions operate throughout life, and are summarized in
Figure 1. The SOX9/SOX8-DMRT1 axis plays an central role in the maintenance of the Sertoli cell fate. Several studies have indicated that SOX9/SOX8 may control
Dmrt1 expression. Sertoli cell-specific ablation of
Sox9/8 at E13.5, shortly after the sex determination stage, leads to a rapid downregulation of
Dmrt1 which is observable just four days later, at E17.5
[30]. In contrast, in mice with a Sertoli cell-specific deletion of
Dmrt1,
Sox9 is downregulated much later, at P14, coinciding with
Foxl2 upregulation
[31]. This suggests that, whereas
Dmrt1 expression seems to be dependent of SOX9,
Sox9 expression is independent of DMRT1 and that the loss of
Sox9 after
Dmrt1 ablation is a secondary consequence of
Foxl2 upregulation. In contrast, other results suggest that DMRT1 might regulate
Sox9 expression as DMRT1 binds near the
Sox9 locus in P28 mouse testes
[31]. In any case, we currently know that the three transcription factors cooperate in the maintenance of the Sertoli cell fate, as Sertoli-to-granulosa transdifferentiation in the postnatal testis is faster and more efficient when
Dmrt1,
Sox9, and
Sox8 are deleted in Sertoli cells, compared to single deletion of either
Dmrt1 or
Sox8/9 alone
[32][33].
A recent study identified genes with Sertoli- and granulosa-biased postnatal expression and showed that many of them were associated with sex-biased differentially-accessible chromatin regions (DARs). In postnatal Sertoli cells, many of the Sertoli-biased DARs were bound by both DMRT1 and SOX9, confirming again postnatal cooperation between the two transcription factors in maintaining the Sertoli cell fate. Furthermore, ChIP-seq analysis of granulosa cells ectopically expressing
Dmrt1 or
Sox9 indicated that DMRT1 and SOX9 jointly bind many sites, although SOX9 was unable to bind most of these sites in the absence of DMRT1, suggesting that during the transdifferentiation process, DMRT1 acts as a pioneer factor promoting chromatin accessibility at regions where SOX9 binds subsequently
[33]. Nevertheless, the fact that ectopic SOX9 expression can reprogram sex-biased gene expression in vitro without activating
Dmrt1 indicates that DMRT1-independent actions of SOX9 also exist during the Sertoli-to-granulosa transdifferentiation process
[33]. Activation of the SOX9/SOX8-DMRT1 axis is essential to maintain
Foxl2 repressed, a process that is mediated by the antagonism of DMRT1 on the feminizing action of retinoic acid (RA)
[31][30]. Two independent genetic cascades are necessary for embryonic and early postnatal maintenance of the female fate, one controlled by WNT signaling and the other by FOXL2
[34][35]. In the adult ovary, FOXL2 alone is essential to maintain the granulosa cell fate, whereas the involvement of WNT genes in female sex maintenance remains to be elucidated
[14]. FOXL2 cooperates with ERα and ERβ in maintaining the granulosa cell fate by directly repressing the
Sox9 promoter
[14][36]. In addition, the action of TGF-β, FST and LATS1/2, RUNX1, FOXO1/3 is necessary for maintaining the granulosa cell fate. Although it is known (1) that FOXL2 can cooperate with members of the TGF-β pathway in maintaining
Fst expression
[37][38]; (2) that FOXL2 is phosphorylated by LATS1, a process that seems to be important for granulosa cell differentiation and follicle maturation
[39]; and (3) that FOXL2 and RUNX1 exhibit overlaps in chromatin binding in fetal ovaries
[40], most of the molecular mechanisms governing the functional relationship among these female promoting genes remain unknown.
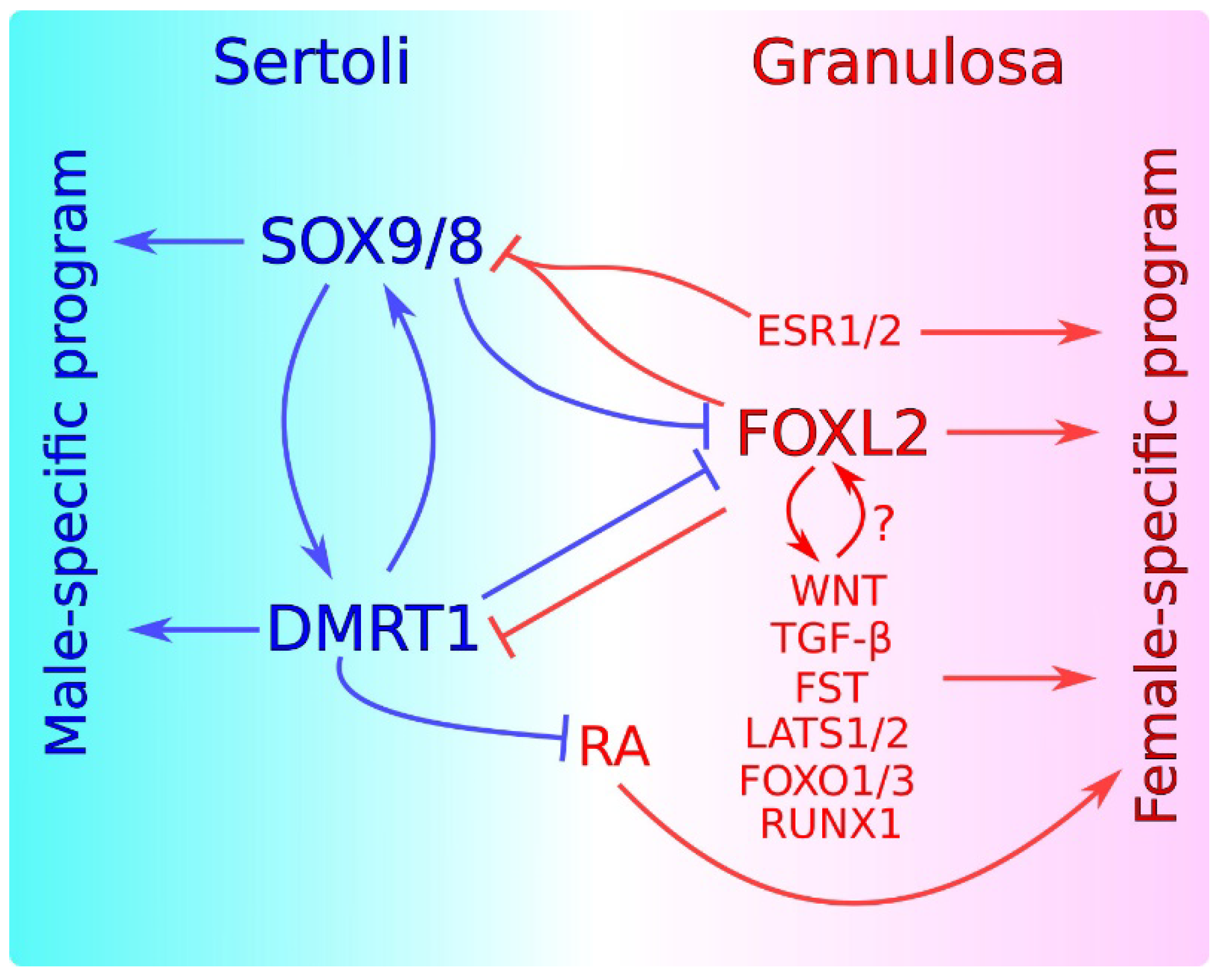
Figure 1. Current model for the maintenance of sex-specific supporting cell fates in adult gonads. In Sertoli cells, SOX9/8 establish a feed-forward regulatory loop with DMRT1, necessary for the maintenance of the male-specific program and for preventing the expression of ovary-promoting genes including FOXL2. DMRT1 inhibits RA signaling, which induces the expression of ovarian genes. In granulosa cells, FOXL2 interacts with ESR1/2 and probably with other genes and molecular pathways including WNT, TGFβ, FST, LATS1/2, FOXO1/3, and RUNX1 to maintain the female-specific program. FOXL2 together with ESR1/2 negatively regulates SOX9/8 and/or DMRT1. Male- and female-promoting genes are in blue and red, respectively. Blue and red lines represent an action exerted by male- and female-promoting genes, respectively. Positive regulation is indicated by arrows. Negative regulation is indicated by perpendicular lines.
Finally, we would like to mention that alterations of the balance between male- and female-promoting factors are associated with gonadal diseases, particularly sex cord tumors. For example, a mutation of FOXL2 (C134W) is found in more than 97% of adult-type granulosa cell tumors
[41]. In addition, other molecules and pathways such as inhibins, TGF-β, WNT, and SOX9 are associated with the pathogenesis or prognosis of gonadal tumors
[42][43][44]. Thus, the exact knowledge of how the sex is maintained is important to understand gonadal function under normal and pathological conditions.
This entry is adapted from the peer-reviewed paper 10.3390/genes12070999